Comprehensive Analysis of Piezoelectric Films
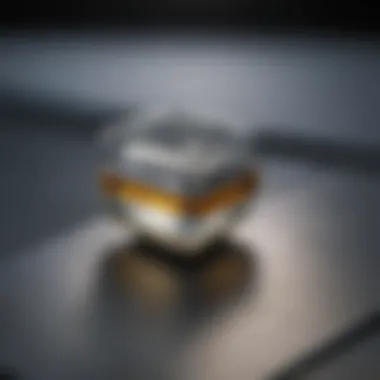
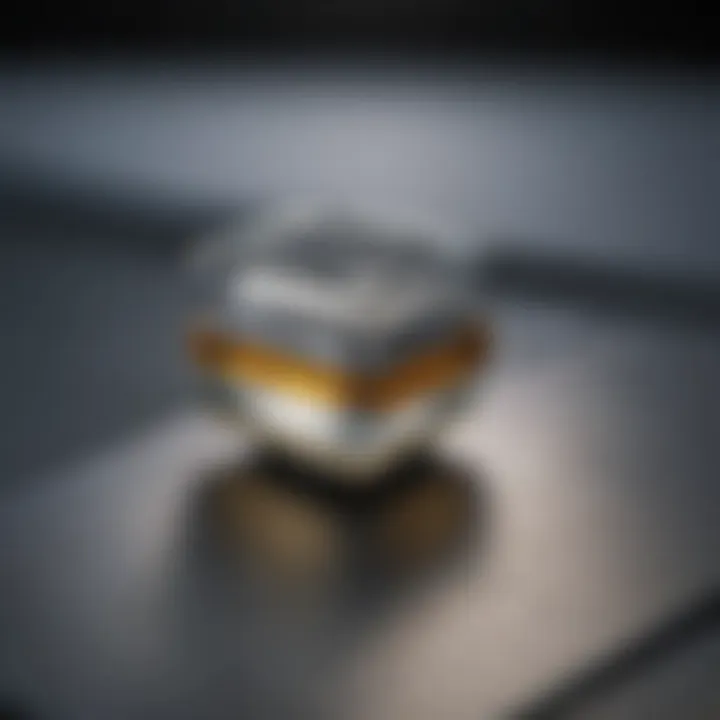
Intro
The field of piezoelectric films holds significant importance in modern technology and research. By understanding the properties of these films, one can appreciate their diverse applications within industries ranging from consumer electronics to aerospace. Due to their ability to convert mechanical stress into electrical energy, piezoelectric materials play a crucial role in a variety of devices. This article will provide a thorough examination of these films, encompassing their unique properties and the various applications in which they thrive.
Understanding piezoelectricity is vital for grasping the relevance of piezoelectric films. The mechanism relies on the alignment of electric dipoles within a material when subjected to mechanical stress. Such a process facilitates the transformation of mechanical energy into electrical signals. This intrinsic property enables a wide range of industrial applications, including sensors, actuators, and energy-harvesting technologies.
In the following sections, we will delve into the research context, methodology, and future directions concerning piezoelectric films. Scholars, researchers, and industry professionals alike will benefit from insight into the emergent technologies and challenges facing this rapidly evolving field.
Prelude to Piezoelectric Film
The exploration of piezoelectric films stands at the intersection of materials science and electrical engineering, drawing significant interest from both academic and industrial sectors. Understanding piezoelectric films is crucial because they possess unique properties that enable conversion between mechanical stress and electrical energy. This characteristic proves invaluable across a range of applications, from sensors to actuators, and impacts various fields such as consumer electronics, healthcare, and energy harvesting.
Definition and Characteristics
Piezoelectric films are thin layers of materials that exhibit piezoelectric properties, meaning they can generate an electrical charge in response to applied mechanical stress. This phenomenon occurs in certain materials, including ceramics and polymers, which can be synthesized or engineered to enhance these properties. Key characteristics of piezoelectric films include:
- Low Weight: Due to their thin nature, piezoelectric films are lightweight and can be integrated into different devices with minimal impact on overall size.
- Flexibility: Many piezoelectric films are flexible, which allows them to conform to various shapes and surfaces. This is particularly advantageous in applications involving wearables or other compact devices.
- Wide Operating Range: Piezoelectric films can function effectively under various environmental conditions, making them suitable for diverse applications ranging from industrial sensors to home automation.
In summary, the definition of piezoelectric films sets the stage for understanding their potential benefits across numerous industries.
Historical Background
In tracing the roots of piezoelectricity, one finds significant milestones that mark its development over time. Initially discovered in the late 19th century, the phenomenon gained recognition when Pierre and Jacques Curie identified it in certain crystals. This foundational work laid the groundwork for later advancements in materials science and technology.
By the mid-20th century, researchers began developing synthetic piezoelectric materials. A notable example is the discovery of polyvinylidene fluoride (PVDF) in 1960, which proved to be an essential polymer in the fabrication of piezoelectric films. Since then, technological advancements have led to the production of diverse materials, leveraging both natural and synthetic sources.
The evolution of piezoelectric film technology continues today, driven by the demand for miniaturized and efficient devices in modern applications. Advances in fabrication techniques allow for the production of increasingly efficient and versatile films. Together, these historical milestones underscore the rich legacy and potential of piezoelectric films in shaping future technological innovation.
Fundamental Principles of Piezoelectricity
Understanding the fundamental principles of piezoelectricity is crucial to grasp how piezoelectric films function and their applications. These principles describe how certain materials can convert mechanical stress into electrical energy and vice versa. This unique characteristic is at the heart of various technological applications, including sensors, actuators, and ambient energy harvesting systems.
Mechanism of Action
The mechanism of action for piezoelectricity involves a direct relationship between mechanical deformation and electrical charge generation. When a piezoelectric material is subjected to a mechanical force, it experiences a change in internal dipole alignment. This change creates a potential difference across the material, generating an electric charge. Conversely, when an electric field is applied, the material deforms physically, leading to mechanical movement. This duality is beneficial in many practical applications where converting energy forms is required.
Types of Piezoelectric Materials
When categorizing piezoelectric materials, they broadly fall into two types: natural and synthetic. Each type carries its own unique properties and applications.
Natural Piezoelectric Materials
Natural piezoelectric materials, such as quartz and certain ceramics, exhibit piezoelectric properties inherently. These materials contribute significantly to the field due to their historical usage and availability. A key characteristic of natural piezoelectric materials is their ability to maintain structural integrity over long periods, making them a reliable choice for various applications. The unique feature of natural piezoelectric materials is their ecological sustainability; they are sourced from nature, reducing the environmental impact associated with sourcing synthetic alternatives. However, they may have limitations in flexibility and size, which can affect their versatility in modern technological applications.
Synthetic Piezoelectric Materials
Synthetic piezoelectric materials, like polyvinylidene fluoride (PVDF) and lead zirconate titanate (PZT), have been engineered for specific applications needing tailored properties. One remarkable characteristic of synthetic piezoelectric materials is their ability to be manufactured with varying thicknesses and compositions, allowing for a wide range of customization. This adaptability makes them a popular choice in advanced applications. Nevertheless, synthetic materials often raise environmental concerns due to their chemical composition and manufacturing processes. With ongoing research, these challenges are being addressed, aiming to develop more eco-friendly options in design and production.
"Understanding the fundamental principles of piezoelectricity not only informs material choice but also guides the future of technological innovations."
Fabrication Techniques for Piezoelectric Films
The methods used to manufacture piezoelectric films are crucial for their performance and application. The quality of the piezoelectric effect heavily relies on the fabrication techniques employed. Understanding these techniques aids in optimizing the films for various uses, such as sensors and energy harvesting devices.
Thin Film Deposition Methods
Thin film deposition methods are primary fabrication techniques utilized in producing piezoelectric films. These methods allow for precise control over film properties, thickness, and composition, enhancing the films' efficiency and functionality.
Physical Vapor Deposition
Physical Vapor Deposition (PVD) is a widely used technique in the fabrication of piezoelectric films. This method involves the physical transfer of material from a solid to a vapor phase and then back to a solid phase on the substrate.
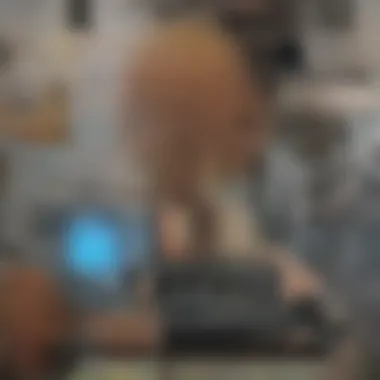
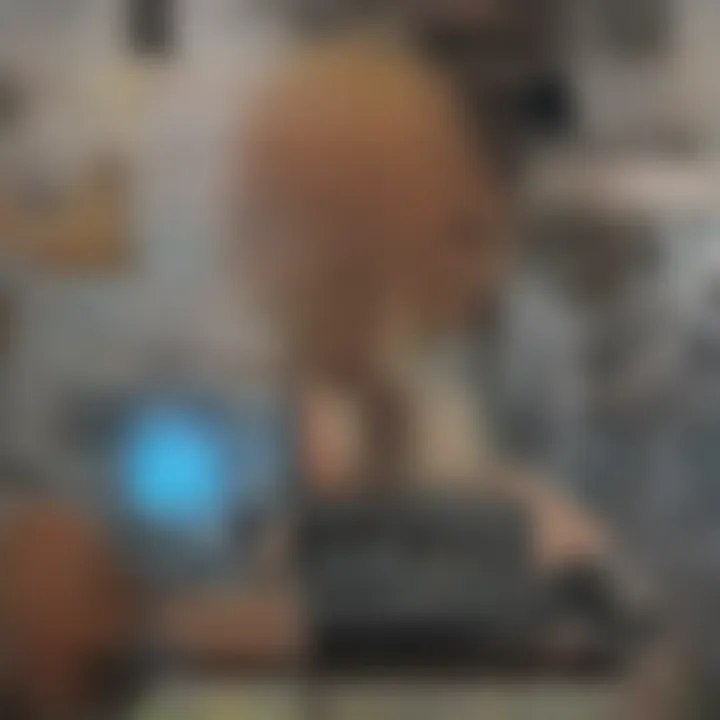
The key characteristic of PVD is its ability to create thin films with high uniformity and purity. This is significant because impurities can adversely affect the piezoelectric properties of the films. PVD is considered a beneficial choice due to its versatility in depositing various materials, like zinc oxide and lead zirconate titanate, which are commonly employed in piezoelectric applications.
One unique feature of PVD is its capability to produce films with controlled stoichiometry, allowing tailored properties conducive for specific applications. The advantages of this technique include the high quality of the deposited films and the ability to easily scale up for industrial production. However, a disadvantage is that the PVD process can be slow and requires a vacuum environment, which may limit its efficiency in some manufacturing scenarios.
Chemical Vapor Deposition
Chemical Vapor Deposition (CVD) represents another essential technique for fabricating piezoelectric films. CVD involves chemical reactions that occur in the gas phase, leading to material deposition on the substrate.
A key characteristic of CVD is its suitability for depositing complex materials with varied compositions. This aspect makes CVD a popular choice for creating piezoelectric films that require specific chemical properties. This technique is particularly advantageous when aiming for films with high adhesion qualities, reducing film delamination risks.
The unique feature of CVD is its ability to create conformal coatings on substrates with complex geometries, which expands the potential applications significantly. Its advantages include homogenous thickness and uniform material properties across large areas. However, it may involve safety concerns regarding the precursors used and the process's operational parameters, which require careful consideration.
Sputtering Techniques
Sputtering Techniques also play a prominent role in the fabrication of piezoelectric films. In this approach, atoms are ejected from a target material and deposited onto a substrate, forming a thin film.
The notable characteristic of sputtering is its high deposition rate and ability to produce films over various sizes and substrates. This flexibility has made sputtering one of the preferred methods in industrial settings. Additionally, the relatively low substrate temperature during sputtering minimizes the risk of thermal damage.
A unique feature of sputtering is its capability to deposit multi-layer structures with distinct compositions, enhancing the piezoelectric characteristics tailored for specific uses. Its advantages include a broad range of materials that can be utilized and the ability to control film thickness precisely. Nevertheless, a potential downside is the equipment complexity and the need for vacuum systems that may drive higher operational costs.
Sol-Gel Processing
Sol-Gel Processing is another pivotal fabrication technique that offers unique opportunities for piezoelectric film production. This method involves transforming a solution (sol) into a solid gel, leading to thin films with desirable properties. It is particularly appreciated for its simplicity and cost-effectiveness. The sol-gel method also facilitates the introduction of different dopants to enhance piezoelectric properties further, making it suitable for research and large-scale production needs. The films produced through sol-gel processing often exhibit improved crystallinity, which is essential for efficient piezoelectric behavior.
Each of these fabrication techniques carries its benefits and challenges. The choice of method often depends on the intended application, material characteristics, and production scale.
Key Properties of Piezoelectric Films
Understanding the properties of piezoelectric films is crucial for grasping their role in various applications and technologies. Key properties define how these films behave under stress and influence their effectiveness in converting mechanical energy into electrical energy, or vice versa. Both electrical and mechanical properties are essential in determining their usability in applications like sensors, actuators, and energy harvesting devices.
Electrical Properties
Electrical properties are vital for the functionality of piezoelectric films. These properties help in understanding how materials respond to electric fields and mechanical stress.
Dielectric Constant
The dielectric constant is a significant aspect when discussing piezoelectric films. It indicates how well a material can store electrical energy in an electric field. A high dielectric constant allows for better energy storage capabilities, which can enhance the overall performance of piezoelectric devices. This is particularly important in applications where efficiency is crucial.
In piezoelectric films, the key characteristic of the dielectric constant ensures that even small applied voltages can produce significant mechanical deformation. This feature makes materials with a high dielectric constant popular in various electronic devices. However, materials with excessively high dielectric constants often exhibit high losses in energy, which can be seen as a disadvantage. Finding a balance is essential for optimal performance.
Piezoelectric Constant
The piezoelectric constant is another critical parameter that directly influences the performance of piezoelectric films. It quantifies the efficiency with which a material converts mechanical stress into electrical energy, and vice versa. In applications like sensors and actuators, a higher piezoelectric constant means a more efficient conversion process, which is beneficial.
One unique feature of the piezoelectric constant is that it varies significantly among different materials. For instance, certain synthetic piezoelectric materials exhibit higher constants compared to natural ones. This variability allows researchers and engineers to choose materials based on the specific needs of their projects. However, higher piezoelectric constants often come with trade-offs in other properties, such as flexibility or temperature stability, requiring careful material selection.
Mechanical Properties
Mechanical properties influence the durability and flexibility of piezoelectric films, determining their practical applications. These properties are particularly critical in environments where the films will experience repeated stress.
Flexibility
Flexibility is a defining characteristic of piezoelectric films that makes them suitable for a variety of applications. A flexible film can bend and stretch without losing performance, allowing it to be integrated into irregular surfaces or wearable technology. This property enables the development of novel devices that can conform to human movements or complex mechanical structures.
However, the flexibility of a piezoelectric film can also come with disadvantages. Some flexible materials may sacrifice mechanical stability or durability, making them less suitable for high-stress applications. Therefore, when selecting materials, the intended use and environmental factors need to be considered to ensure the right balance of flexibility and strength.
Strength
Strength is another critical mechanical property of piezoelectric films. It determines how well a film can withstand external forces without breaking or deforming. A strong piezoelectric film is necessary for applications in harsh environments, where mechanical stress can frequently occur.
The key characteristic of strength is interconnected with the overall performance of these films. For instance, a strong but less flexible piezoelectric film is ideal for static applications where movement is minimal. Conversely, in dynamic environments, a compromise may be necessary to enable both strength and some degree of flexibility. Understanding these trade-offs is vital when developing new technologies that utilize piezoelectric films.
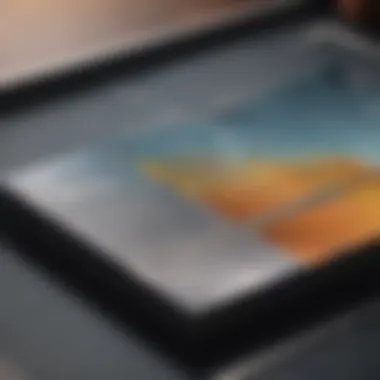
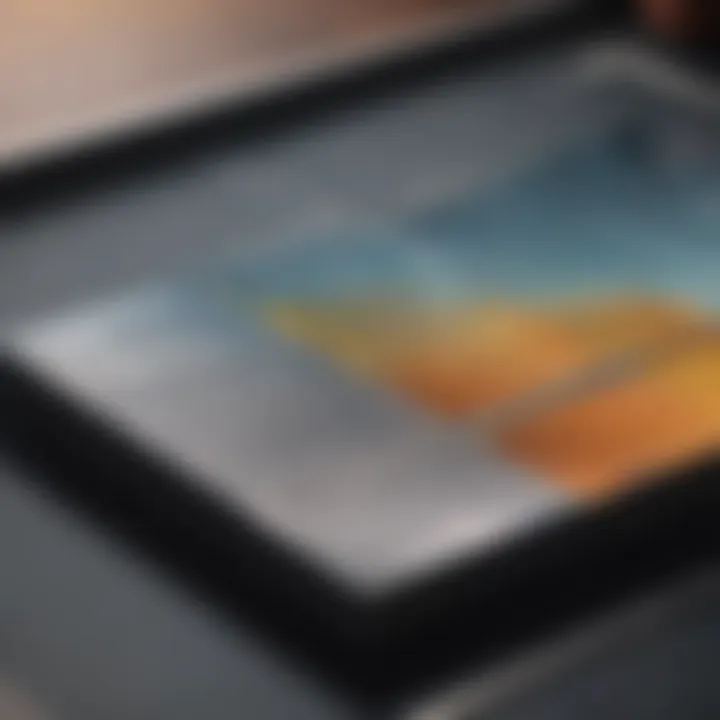
Key properties of piezoelectric films, including electrical and mechanical characteristics, significantly influence their potential applications in various fields.
In summary, the key properties of piezoelectric films play a pivotal role in determining their functionality and suitability for specific applications. A clear comprehension of these properties allows for informed material selection and innovative applications in electronics and materials science.
Applications of Piezoelectric Films
Piezoelectric films have gained significant attention due to their versatile applications across various sectors. Their unique ability to convert mechanical energy into electrical energy—and vice versa—renders them ideal candidates for numerous technology advancements. Understanding these applications sheds light on their importance in both current and future technological landscapes.
Sensors
Pressure Sensors
Pressure sensors are crucial in various industries such as automotive, aerospace, and healthcare. They measure force or pressure changes, translating these mechanical forces into electrical signals. A key characteristic of pressure sensors is their high sensitivity, making them a preferred choice in environments where precise measurements are essential.
One unique feature of pressure sensors made from piezoelectric films is their rapid response time. This characteristic allows for real-time monitoring and immediate feedback, which is essential in safety applications. However, these systems can be susceptible to noise and require careful design to mitigate such issues.
Medical Sensors
Medical sensors utilizing piezoelectric films have revolutionized diagnostics and patient monitoring. These sensors can detect various signals, including physiological parameters such as blood pressure and heart rate. Their compact size and integration capability with other devices make them highly beneficial in medical applications.
A key advantage of medical sensors is their non-invasiveness. They can conduct measurements without any physical intrusion, which greatly enhances patient comfort. Nonetheless, the accuracy of these sensors can vary based on various external factors, including temperature and environmental conditions.
Actuators
Micropositioning Systems
Micropositioning systems are critical in precision engineering. They require minimal movement to achieve accurate positioning, often necessary in fields like optics and semiconductor manufacturing. Piezoelectric films are advantageous in these systems due to their ability to provide fine control with rapid execution.
The ability to create small movements with high precision is a significant characteristic of micropositioning systems. This feature makes them a popular choice for applications where even slight deviations can lead to performance degradation. However, like any technology, they have limitations in terms of range and may require complex control systems.
Robotic Applications
Robotics represents another significant application area for piezoelectric films. They are often incorporated as actuators in robotic systems, enabling precise motion control. A standout feature is their ability to generate high forces relative to their size, making them practical for compact robotic designs.
The agility and responsiveness of piezoelectric actuators in robotics is a critical advantage. However, these systems may face challenges related to energy efficiency, raising concerns about the energy consumption in long-term applications.
Energy Harvesting
Power Generation Techniques
Power generation techniques related to piezoelectric films are particularly appealing in the context of sustainable energy. They can harvest energy from ambient vibrations or movements, converting mechanical energy into electrical energy effectively. This application is vital for powering low-energy devices without reliance on traditional power sources.
Key characteristics of these power generation methods include low maintenance and long operational lifespan. They provide an environmentally friendly approach to energy generation. Nevertheless, they typically offer lower energy outputs, which might limit their application in high-power scenarios.
Applications in Wearable Technology
Wearable technology is another promising area for piezoelectric film applications. These technologies often utilize energy harvested from physical activity. The adaptability of piezoelectric films allows them to function in compact devices worn on the body without significantly adding to their weight.
The unique feature of these applications is their seamless integration with user activities, enabling continuous monitoring without requiring additional energy sources. Despite the advantages, the durability of these devices can be a concern, particularly under rigorous physical conditions.
Piezoelectric films serve essential roles in advancing sensor technology, actuator systems, and energy harvesting applications, reflecting a notable intersection of innovation and functionality.
Challenges in Piezoelectric Film Technology
The advancement of piezoelectric film technology holds significant promise, yet it is accompanied by distinct challenges that need addressing. Understanding these challenges is crucial for both current research and future applications. Two main categories of these challenges are material limitations and manufacturing constraints. Each plays a critical role in the development, performance, and scalability of piezoelectric films.
Material Limitations
The performance of piezoelectric films greatly depends on the inherent properties of the materials from which they are made. Common materials include polyvinylidene fluoride (PVDF) and certain ceramics. However, these materials come with limitations. For instance:
- Temperature Sensitivity: Many piezoelectric materials perform poorly at extreme temperatures. This restricts their application in environments that undergo wide temperature fluctuations.
- Mechanical Properties: While flexibility is desirable, some materials lack the necessary mechanical strength to withstand operational stress. This can lead to premature failure.
- Fabrication Challenges: Finding suitable materials that can be manipulated without losing their piezoelectric properties can be difficult. The synthesis process may introduce defects that degrade performance.
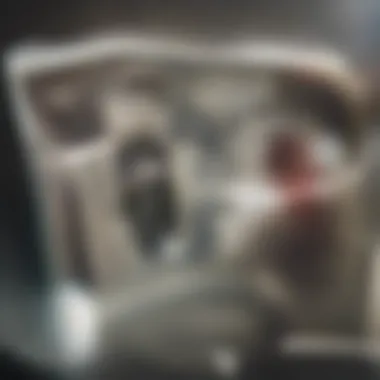
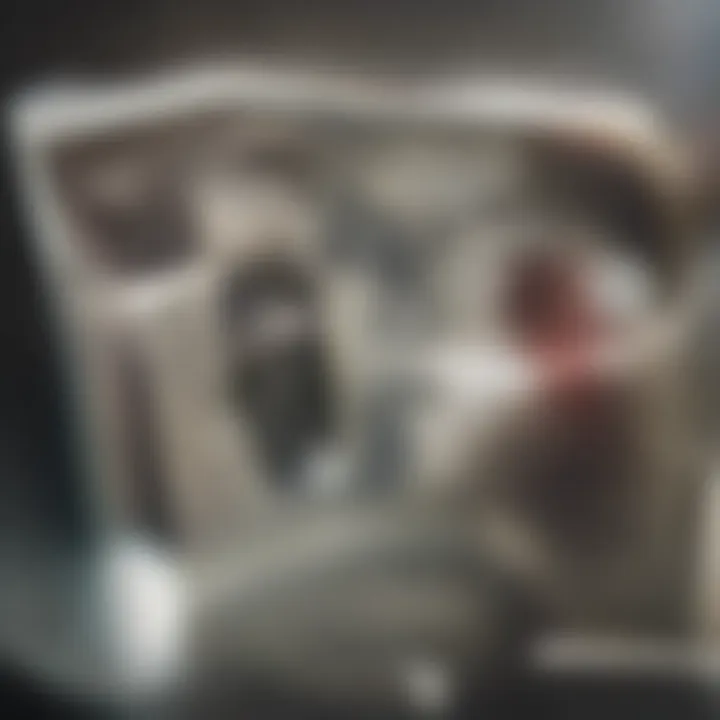
Understanding these material limitations allows researchers to focus on developing new, innovative materials that can overcome these issues. This might include exploring composite materials or improving existing ones to enhance their operational range and efficiency.
Manufacturing Constraints
Manufacturing piezoelectric films involves intricate processes that can be economically and technically challenging. Common constraints in manufacturing include:
- Scalability Issues: Producing high-quality piezoelectric films at scale is difficult. Techniques suitable for lab settings often do not transfer effectively to large-volume manufacturing.
- Cost Factors: The cost of materials and the machinery required for production can pose significant barriers. Lower production costs are essential to increase competitiveness in the market.
- Consistency and Quality Control: Ensuring uniform quality in each batch of films is crucial. Variations in the manufacturing process can lead to inconsistencies in film performance.
Efforts to mitigate these constraints focus on refining fabrication processes and employing automation where possible. Adjusting techniques and materials in alignment with industry demands is necessary for advancing piezoelectric film technology.
"Innovation in material science is key to overcoming the limitations of piezoelectric technology."
Both material limitations and manufacturing constraints present hurdles that need to be navigated for the technology to thrive. Ongoing research and development in these areas will play a pivotal role in shaping the future landscape of piezoelectric films.
Future Directions in Piezoelectric Film Research
The investigation of piezoelectric films is not just about understanding their current uses; it also entails exploring the vast potential ahead. This section emphasizes innovative methodologies and cutting-edge applications that might shape the future of piezoelectric technology. Attention towards future directions ensures a continuous evolution in materials science and various engineering streams. The integration of novel materials and advanced applications is essential to harness the fullest capabilities of piezoelectric films.
Innovative Materials
Composite Materials
Composite materials have gained traction in piezoelectric film research. These materials combine two or more constituents, often resulting in superior properties compared to their individual components. The key characteristic of composite materials is their ability to merge the strengths of diverse materials for enhanced functionality. Their lightweight nature and improved mechanical flexibility make them particularly attractive choices for applications requiring durability without added weight.
One unique feature is the customizable nature of composites. Different combinations of materials allow researchers to fine-tune electrical and mechanical properties. The main advantage is the enhancement of piezoelectric efficiency, which opens possibilities for various applications ranging from micro-sensors to energy harvesting devices. However, potential challenges include complicated manufacturing processes and variability in properties depending on the composition.
Nanoscale Films
Nanoscale films represent another promising area of future research in piezoelectric films. These films, with thicknesses on the nanometer scale, exhibit unique electrical and mechanical characteristics. A standout feature is their high surface area-to-volume ratio, which amplifies piezoelectric responses. The reason for their growing popularity is their applicability in miniaturized technology, particularly where space and weight are critical factors.
The unique performance of nanoscale films makes them suitable for advanced electronic components and sensors in highly integrated systems. Significant advantages include the potential for lower energy consumption and faster response times. Nevertheless, challenges persist, such as difficulties in large-scale production and material stability over time.
Advanced Applications
Smart Textiles
Smart textiles incorporate piezoelectric films to create fabrics that can respond to environmental stimuli. This aspect is essential for wearables that monitor biological signals. The notable characteristic of smart textiles is their flexibility; they can conform to body movements while providing important data. The integration of these materials offers a beneficial turn in healthcare and fitness monitoring.
A unique feature is their potential for real-time feedback, enabling applications in active lifestyles. The primary advantage lies in their inherent ability to combine aesthetics with functionality. However, durability over time and the integration of electronics pose challenges that need to be considered within the development of these technologies.
Biomedical Devices
Biomedical devices leveraging piezoelectric films represent a revolutionary direction for healthcare technology. These devices can convert mechanical stress from body movements into electrical signals, providing new methods for diagnostics and treatment. A key characteristic is the biocompatibility of specific piezoelectric materials used, which is crucial for implantation and long-term contact in the body.
The unique feature of piezoelectric biomedical devices is their ability to generate energy from physiological movements, creating self-powering systems. The advantages are noteworthy, as these devices can enhance patient experience and streamline data collection. On the downside, regulatory challenges and the need for thorough testing must be addressed before widespread adoption.
Future research in piezoelectric films focuses on innovation in materials and applications, paving the way for significant advancements in diverse fields.
Culmination
In this article, we've explored the intricate landscape of piezoelectric films. The discussion ranged from their unique properties to the vast array of applications in various sectors, such as sensors, actuators, and energy harvesting. The importance of understanding these films extends beyond academic curiosity; it has practical implications for technology and industry.
Summary of Insights
Piezoelectric films exhibit key characteristics that make them invaluable in modern applications. Their ability to convert mechanical stresses into electrical energy and vice versa is notable. This property derives from the crystal structures of piezoelectric materials, including both natural and synthetic types. Furthermore, the manufacturing methods discussed, such as thin film deposition and sol-gel processing, reveal the sophistication required to create these advanced materials.
Additionally, we analyzed their respective electrical and mechanical properties, emphasizing flexibility and robustness. The insights gained from examining current applications offered a clear picture of piezoelectric technology's role in fields like robotics and wearable tech. However, several challenges exist, from material limitations to manufacturing constraints, which must be addressed to advance the field.
Implications for Future Research
Looking forward, the prospects for piezoelectric film research are promising yet complex. There is significant interest in developing innovative materials, including composites and nanoscale films, which may enhance performance and broaden applications. Furthermore, advanced applications in smart textiles and biomedical devices stand to significantly impact both consumer technology and healthcare.
Further investigation into improving the efficiency, durability, and integration of piezoelectric materials can lead to groundbreaking advancements. Researchers must focus on overcoming existing challenges while exploring new realms of application. The synergy between academic research and industrial application is essential for fostering innovations.
In summary, as piezoelectric film technology continues to evolve, its contributions to various sectors will not only bring about advancements in device functionality but will also promote sustainable energy solutions. For students, researchers, and professionals, understanding and engaging with this field holds the potential for meaningful impact in technology and society.
The intersection of innovation and tradition in piezoelectric research promises an exciting path forward for both academia and industries.