Assessing Oxygen Levels: Methods and Their Implications
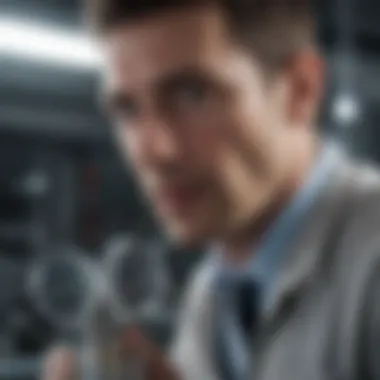
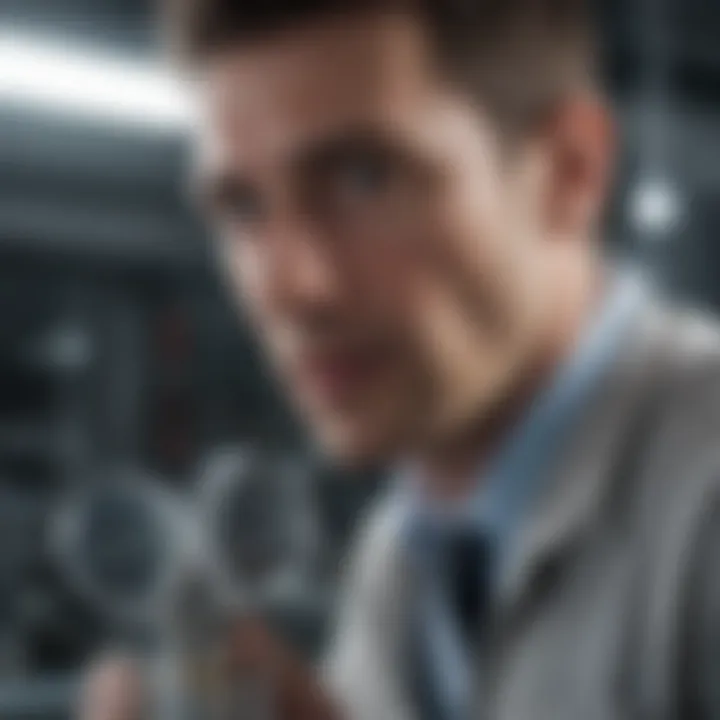
Intro
Accurate measurement of oxygen levels plays a crucial role across multiple sectors, including healthcare, environmental science, and various industries. Oxygen, being a fundamental element for life and combustion, presents unique challenges when assessing its levels—challenges that can lead to grave consequences if not addressed properly.
In medical settings, for instance, monitoring oxygen saturation in patients can mean the difference between life and death. Without precise measurements, healthcare professionals risk making decisions that could severely affect patient outcomes. This explains why devices like pulse oximeters and arterial blood gas analyzers have become staples in the medical toolkit. Their accurate functioning is paramount, as it ties directly to patient safety.
On the other hand, environmental monitoring demands the assessment of oxygen levels in ecosystems to study biodiversity and climate change. Too much or too little oxygen can have catastrophic effects on aquatic life and the overall health of our planet. This brings us to industrial settings where oxygen is vital in processes like welding and metal cutting. Here, not only is the level of oxygen critical for operations, but it also directly impacts workers' safety.
Given the multi-dimensional importance of oxygen measurements, this article will dive into the methodologies currently employed, shedding light on their respective advantages and drawbacks. Furthermore, we’ll explore recent advancements in technology, highlighting how they promise to revolutionize oxygen monitoring—a needed elevation in a diverse array of applications.
Foreword to Oxygen Measurement
Understanding how to measure oxygen levels is not just a technical necessity; it serves a pivotal role across various fields. The significance of oxygen, a vital element for life, cannot be overstated. With its molecular makeup dictating how living organisms thrive, the measurement of oxygen levels provides insights essential for health, safety, and environmental sustainability.
The Importance of Oxygen Monitoring
Keeping tabs on oxygen levels is akin to keeping an eye on the temperature gauge in a car; it's crucial for smooth operation. In healthcare, for instance, monitoring oxygen saturation can help detect early signs of respiratory distress. A dip in oxygen levels might indicate underlying issues, prompting timely medical intervention. In industrial settings, where processes hinge on optimal oxygen concentrations—like in welding or metal fabrication—accurate measurements avert potential accidents, ensuring both worker safety and product quality.
In addition to health and safety implications, oxygen monitoring contributes significantly to environmental assessments. Elevated or reduced oxygen levels can indicate pollution or ecosystem imbalances, necessitating swift action or further analysis.
Overview of Applications
The applications of oxygen measurement span a broad spectrum:
- Healthcare: Devices like pulse oximeters are commonplace in clinical environments, allowing for continuous monitoring of patients' statuses, particularly those with chronic lung conditions.
- Environmental Science: Researchers utilize various techniques to measure dissolved oxygen in waterways, an indicator of aquatic health alongside the effects of pollution.
- Industrial Applications: Oxygen levels are monitored during combustion processes, where appropriate levels ensure efficiency and safety.
Each field utilizes specific methodologies tailored to their unique requirements, but the underlying theme of monitoring oxygen remains consistent across the board. Ensuring oxygen levels are within ideal ranges stands as a cornerstone of operational efficacy in multiple contexts.
Fundamental Concepts of Oxygen
Understanding the fundamental concepts of oxygen is vital for several reasons. It serves not just as a foundation for measuring oxygen levels but also as a stepping stone to unravel the complexities behind various applications. Having a solid grip on these concepts ensures that researchers and practitioners can accurately interpret data, thereby influencing both clinical practices and industrial standards.
Nature of Oxygen
Oxygen, with the chemical symbol O, is an element that is indispensable for life as we know it. It exists in a gaseous state at room temperature and is odorless and colorless. Its atomic structure comprises eight protons, eight neutrons, and eight electrons, giving it unique properties.
It is found abundantly in nature, predominantly forming in diatomic molecules (O2). This diatomic form is what most organisms rely on for metabolic processes. Interestingly, oxygen is also highly reactive, combining with nearly all elements and forming various compounds, including water and organic materials.
In a practical sense, oxygen's presence in the atmosphere is crucial. Here are some interesting facts about its nature:
- Distribution: Comprising about 21% of the Earth's atmosphere, oxygen is essential for combustion, respiration, and many industrial processes.
- Solubility in Water: Its solubility in water is critical for aquatic life as it enables fish and other organisms to breathe underwater.
- Role in Combustion: Oxygen is a key player in combustion reactions, making it vital in fuel processes and energy production.
Physiological Role in Living Organisms
Oxygen's role in living organisms goes beyond simple respiration. It is intricately linked with processes that sustain life across the biosphere. At a cellular level, oxygen plays a crucial role in metabolism, influencing how cells generate energy. This energy is pivotal for all cellular processes, allowing organisms to grow, reproduce, and adapt.
The most significant biological process that utilizes oxygen is cellular respiration. Here’s a closer look at its implications:
- Energy Production: In mammals, aerobic respiration, which occurs in the mitochondria, is the process that extracts energy from glucose using oxygen. Without oxygen, cells rely on anaerobic processes, yielding less energy and producing byproducts like lactic acid.
- Biosynthesis Pathways: Oxygen also participates in various biosynthetic pathways, facilitating the production of molecules needed for cellular structure and function.
- Signaling Pathways: Moreover, oxygen levels can influence gene expression and cellular signaling, impacting overall health and disease states.
Oxygen is not merely about sustenance—it's a dynamo driving the very engines of life.
In summary, understanding the fundamental characteristics and roles of oxygen not only enhances our grasp of biological and environmental processes but also informs the methodologies employed in various fields, ranging from healthcare to industrial applications. Recognizing the importance of oxygen sets the stage for accurate measurement techniques and underscores its critical role in sustaining life.
Methods of Measuring Oxygen Levels
Measuring oxygen levels is critical for various disciplines, from healthcare to environmental science to industrial applications. The methods employed to gauge oxygen content influence the accuracy of monitoring and measurement, which in turn impacts safety protocols, health assessments, and research outcomes. Understanding these methods is essential, as each technique has its own strengths and weaknesses that can affect data reliability.
Pulse Oximetry
Principles of Operation
Pulse oximetry stands out for its ability to provide real-time data on oxygen saturation non-invasively. This method uses a light source and photodetector, typically attached to a fingertip, to determine the percentage of oxygenated hemoglobin in the bloodstream. The key characteristic of this method lies in its simplicity and speed, which makes it particularly popular in clinical settings.
The unique feature of pulse oximetry is the ability to operate without drawing blood—a significant advantage for patient comfort and rapid assessments. However, it does have limitations, such as reduced accuracy in cases of poor circulation or skin pigmentation.
Advantages and Disadvantages
The primary advantage of pulse oximetry is its non-invasive nature, allowing for quick and continuous monitoring of patients. This method quickly alerts medical personnel to any changes in oxygen saturation, which is crucial in emergency situations.
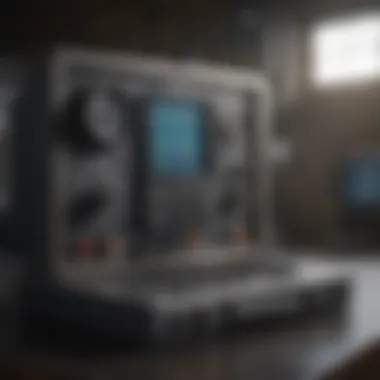
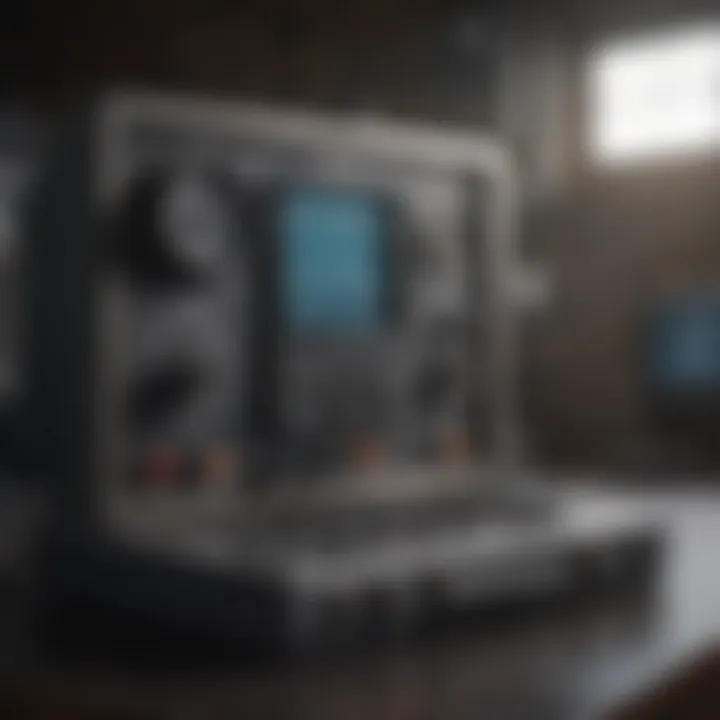
However, while pulse oximetry is widely trusted, it’s not foolproof. Factors like nail polish, artificial nails, or ambient light can interfere with readings. Critics argue its reliability can be compromised under certain conditions.
Special Considerations
In any application of pulse oximetry, integrity of the sensor and proper placement are pivotal. Issues like motion artifacts may skew results during measurement, leading to potentially faulty outcomes. A notable consideration is recognizing that while pulse oximetry is excellent at measuring oxygen saturation, it does not assess carbon dioxide levels, which can also be important in critical care settings.
Arterial Blood Gas Analysis
Procedure and Equipment
Arterial blood gas analysis is a more invasive method that provides a comprehensive evaluation of oxygen and carbon dioxide levels in the blood. This procedure typically involves accessing an artery, often in the wrist, to draw a blood sample.
The equipment needed includes syringes designed for blood gas sampling and analyzers that can quickly interpret the sample. A key characteristic of this method is the detailed insight it offers into metabolic and respiratory function, making it invaluable in intensive care and critical situations.
One downside of this method is that it requires trained personnel, which can pose challenges in resource-limited settings.
Clinical Significance
The clinical significance of arterial blood gas analysis cannot be overstated. It provides essential information necessary for diagnosing conditions such as acidosis or alkalosis. The ability to detect carbon dioxide retention or respiratory failure is also critical in guiding treatment strategies.
Nonetheless, its invasiveness poses comfort issues for patients and can introduce potential complications, including bleeding or infection.
Limitations and Risks
Every method has its pitfalls, and arterial blood gas analysis is no exception. The primary limitation is its invasiveness. Patients may experience pain or anxiety, which can complicate the process. Furthermore, not all healthcare settings may have the capabilities for rapid analysis, delaying critical information that could affect patient outcomes.
Electrochemical Sensors
Types of Sensors
Electrochemical sensors present a diverse range of devices tailored specifically for monitoring oxygen levels in various environments. These sensors typically employ an electrochemical reaction to evaluate the concentration of oxygen. The notable characteristic of electrochemical sensors is their portability, allowing for field applications where traditional measurement methods fall short.
A unique feature here is the flexibility of use; they can be designed for specific environments, from underwater exploration to industrial air quality monitoring. However, careful calibration and maintenance are essential to ensure accurate readings and prolong the sensor’s lifespan.
Operational Mechanisms
The operational mechanisms of electrochemical sensors depend on the type of reaction they utilize. Most commonly, they involve a two-electrode or three-electrode system where oxygen is reduced at the electrode, generating a measurable electric current that corresponds to the oxygen concentration. This fundamental mechanism highlights their capability to deliver real-time data effectively.
However, sensitivity to other gases can be a hindrance, as they might produce misleading results if not designed with appropriate filters.
Field Applications
Electrochemical sensors find their niche in various field applications. In industrial settings, they are crucial for ensuring safe working conditions by constantly monitoring oxygen levels in the environment, from welding sites to confined spaces. The ability to quickly detect and report unusual oxygen levels serves as a vital safety measure.
But these sensors are not infallible. Their performance can be influenced by factors such as temperature and humidity, making it essential to select models helpful in specific environmental conditions.
Optical Measurement Techniques
Fiber Optic Sensors
Fiber optic sensors are indeed a game-changer in the realm of oxygen measurement. They leverage the unique properties of light to evaluate oxygen levels, transmitting signals through optical fibers. This method can measure oxygen in challenging environments, making it very beneficial for research and monitoring in the environmental sector.
The standout feature of fiber optic sensors is their immunity to electromagnetic interference, allowing for precise measurements in industrial settings where other techniques may fail. Still, their complexity can pose challenges in calibration and installation in some cases.
Fluorescent Probes
Fluorescent probes operate by detecting changes in fluorescence when oxygen levels fluctuate. This technique is particularly advantageous in biological and environmental research, providing insights into processes like cellular respiration or water quality assessments.
A unique characteristic of fluorescent probes is their ability to operate at a cellular level, making them instrumental in research that requires high sensitivity. However, they do depend heavily on specific environmental light conditions for optimal performance, which can hinder effective usage in certain scenarios.
Measurement Range and Accuracy
The measurement range and accuracy of optical techniques are generally superior to some more traditional methods, allowing for detailed and nuanced readings. Fiber optic and fluorescent systems can detect minuscule changes in oxygen levels.
But with these benefits come challenges; complex setups and the need for skilled personnel to interpret data can limit their usability, particularly in resource-strapped environments.
Other Emerging Technologies
Nanotechnology-Based Sensors
Nanotechnology-based sensors represent the forefront of innovation in oxygen level measurements. These sensors utilize nanomaterials to detect oxygen, greatly enhancing sensitivity and speed through superior surface-to-volume ratios. This technology holds promise across various sectors, especially healthcare and environmental monitoring.
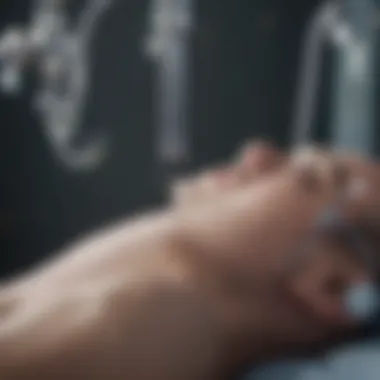
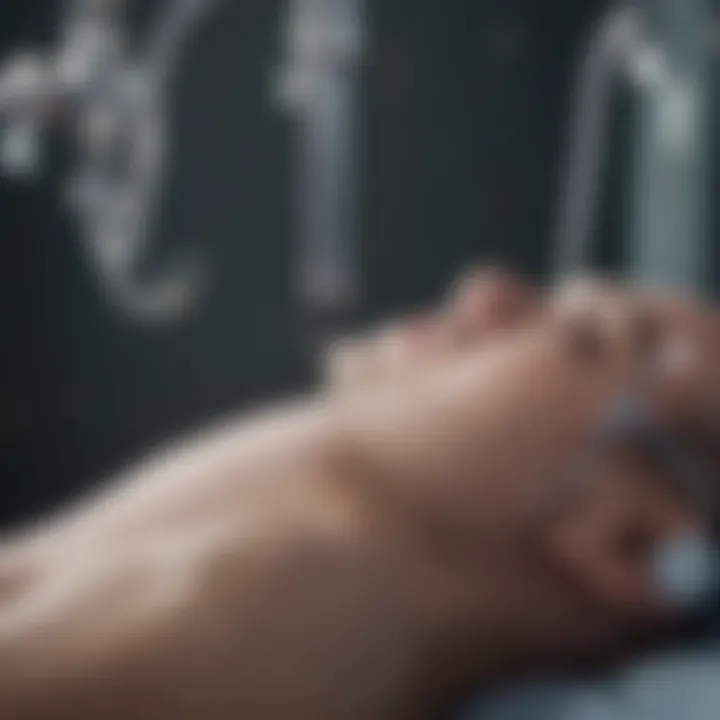
A compelling feature is their miniaturization, capable of integrating into wearable medical devices, enhancing patient monitoring capabilities. Yet, the technology is still developing, raising concerns about long-term reliability and cost-effectiveness.
Remote Sensing Applications
Remote sensing applications in oxygen measurement are reshaping our understanding of environmental health across geographic extents. This technology allows scientists to gauge oxygen levels from satellites and aerial platforms, marking a significant leap forward in atmospheric science.
A notable aspect of this application is the scale at which measurements can be taken, enabling large-scale assessments that ground-based methods cannot achieve. However, data interpretation can be complex, requiring robust analytical tools to gather actionable insights.
Future Prospects
Looking ahead, future prospects in oxygen measurement methods are very promising. Continued advancements in sensor technology, especially in nanotechnology, will likely produce devices that are not only smaller but also more precise and cost-effective.
This innovation will enable more widespread use across various sectors, enhancing our ability to monitor and respond to oxygen levels effectively. Such developments could prove transformative in areas such as preventive healthcare and environmental management, ensuring sustained efforts to maintain the delicate balance of oxygen in our lives.
Evaluating Measurement Accuracy and Reliability
Measuring oxygen levels isn't just a matter of slapping a sensor together and calling it a day. In any given scenario—be it in a hospital, an industrial setting, or out in nature—accuracy and reliability of these measurements are pivotal. If the readings are off, the consequences can range from mere inconvenience to serious health risks, or even disasters.
Calibration Procedures
Calibration acts as the bedrock of accurate oxygen measurement. It involves adjusting the device to ensure that its output aligns with a known standard. This procedure typically starts with a baseline reference, often using a gas with a specific concentration of oxygen. Think of it like tuning a musical instrument; if it’s not calibrated right, everything else will sound off.
The process is as follows:
- Preparation: Gather needed materials such as calibration gases or standard solutions.
- Setup: Connect the oximeter or sensor to the calibration equipment. This requires familiarity with how each device interfaces.
- Adjustment: Slowly introduce the calibration gas to the sensor and adjust it until the displayed reading matches the known concentration.
- Documentation: Keep a record of calibration dates, conditions, and results to track any drift over time. This not only helps in troubleshooting but also builds a history for audits or reviews.
Regular calibration lends a hand in making the readings consistent and trustworthy. Without it, even the most sophisticated equipment can turn into a shot in the dark.
Quality Assurance in Measurement
Quality assurance is the umbrella under which calibration falls. It encompasses the entire process of measuring oxygen, from design to execution, to ensure that each measurement is as precise as it can be. Having robust QA protocols can make a significant impact by reducing potential errors. This can involve:
- Routine Checks: Regular testing of measurement systems helps identify anomalies early on.
- Environmental Controls: Factors like temperature and humidity can skew readings, so maintaining controlled conditions is vital.
- Staff Training: Ensuring that personnel understand the systems in place adds a human dimension to quality assurance. Trained staff identify issues quicker and apply necessary corrections promptly.
Quality assurance isn’t a one-and-done deal. It’s an ongoing commitment.
The importance of dignified quality assurance can't be overstated. It not only safeguards health and safety but also promotes confidence in environmental studies or industrial processes where accurate measurements are indispensable.
Implications of Oxygen Level Measurements
Understanding the implications of oxygen level measurements is crucial not just for academic discourse but also for practical applications across various fields. Knowing how to accurately gauge oxygen levels can affect health outcomes, inform regulatory policies, and influence environmental strategies. With the backdrop of ongoing concerns about air quality and patient health, the stakes are higher than ever.
Precise measurement techniques serve as the backbone for making informed decisions in healthcare, environmental science, and industry. Each context presents unique challenges and exigencies, underscoring the need for specialized methodologies. The implications stretch beyond mere analytics—improving quality of life, enhancing safety protocols, and addressing climate-related issues are some key facets that emerge.
Healthcare Applications
Monitoring Chronic Conditions
Monitoring chronic conditions, such as COPD or asthma, hinges on understanding a patient's oxygen levels. This process is a game-changer in managing and potentially improving patient outcomes. Utilizing technologies like pulse oximeters, healthcare professionals consistently track oxygen saturation, allowing for timely interventions. The key characteristic here is real-time monitoring, which means patients do not have to rely solely on periodic visits to the doctor for evaluations. This accessibility can foster better self-management of chronic ailments.
The unique feature of this monitoring is its versatility. From remote patient monitoring in telehealth setups to at-home monitoring, it provides a safety net for patients with long-term conditions. Moreover, the advantage lies in its non-invasive nature, making it a popular choice. On the flip side, occasional inaccuracies can occur due to external factors such as skin pigmentation or motion, which is a limitation to be mindful of.
Emergency Response Situations
In emergency medical situations, rapid assessment of oxygen levels becomes pivotal. First responders often utilize portable pulse oximeters to ascertain a patient’s immediate needs. The key characteristic here is speed. Quick readings can guide decisions about supplemental oxygen or other treatments, impacting patient survival and recovery trajectories.
The unique aspect of this application is its immediate relevance in life-or-death situations. When a patient is in distress, every second counts. The benefit of being able to make prompt judgments is crucial, but it's not without downsides. These handheld devices may occasionally misread in chaotic environments or may be affected by factors like nail polish or cold extremities, introducing elements of uncertainty.
Environmental Monitoring
Air Quality Assessment
Air quality assessment is a prominent application of oxygen level measurements. Identifying pollutants and understanding air composition is essential for public health and urban planning. The key characteristic of air quality assessments is their rigorous data collection methods. Instruments like gas analyzers and air sampling devices do the heavy lifting in this domain, providing invaluable data for studies and regulatory measures.
A distinct feature of air quality assessments is their ability to inform policy initiatives. Government bodies harness this data to develop regulations aimed at reducing air pollution. While the benefits are clear, resources, time, and financial implications can pose challenges. Often, comprehensive assessments require extensive networks of sensors, which can be expensive and laborious to maintain.
Impact on Climate Studies
Climate studies increasingly rely on accurate oxygen level measurements to understand atmospheric changes and their downstream effects on ecosystems. The key characteristic of these studies is their long-term analysis, requiring sustained monitoring over time. Understanding oxygen fluctuations can unveil insights into carbon cycles and climate change impacts.
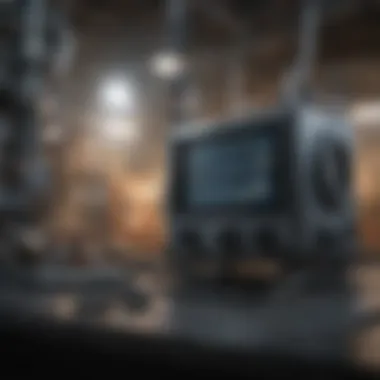
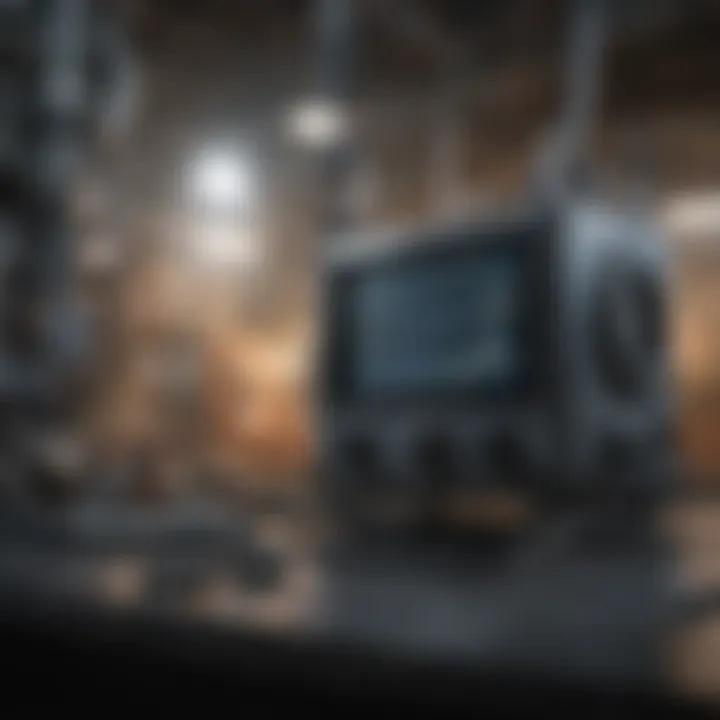
One standout feature of this application is interdisciplinary collaboration, as climatologists often work with ecologists and atmospheric scientists to paint a holistic picture. While these studies are beneficial for theoretical understanding and policy advocacy, they can suffer from gaps in data continuity, primarily when funding dips or logistical issues arise.
Industrial Applications
Safety Protocols in Manufacturing
Safe manufacturing processes heavily depend on oxygen level monitoring. In industries where flammable materials are handled, ensuring oxygen levels are within safe limits can prevent catastrophic incidents. The key characteristic of safety protocols lies in their preventive nature, prioritizing worker safety and compliance with regulations.
The unique edge here is how rules are enforced via continuous monitoring, creating a safer work environment. But challenges do arise; sometimes the sensors may not be calibrated correctly, leading to false alarms or oversights, both dangerous in such settings.
Process Optimization
In optimizing manufacturing processes, accurate oxygen measurement can increase efficiency and product quality. This application focuses on using oxygen levels to tweak processes in real time. The key characteristic here is adaptability—being able to adjust processes based on immediate feedback can significantly impact costs and output quality.
One unique advantage of this is the potential for innovation in product development as companies utilize data-driven decisions to enhance their offerings. However, risks remain; reliance on specific metrics might lead to neglecting other crucial factors, hence a balanced approach is essential to avoid pitfalls.
Challenges and Limitations in Measurement
Accurate measurement of oxygen levels is paramount across various sectors, from clinical settings to environmental monitoring. However, challenges and limitations in this process can significantly impact outcomes. Understanding these issues not only aids in selecting the right technique but also in interpreting results accurately. Therefore, delving into the challenges of oxygen measurement unveils critical elements vital for effective monitoring and decision-making.
Common Sources of Error
Oxygen measurement is fraught with potential inaccuracies. Here are a few prevalent sources of error:
- Calibration Issues: Regular calibration must be performed to ensure measurements are reliable. A failure to do so can lead to incorrect readings, which can have dire consequences, especially in healthcare.
- User Techniques: Improper handling or application of sensors can distort results. For instance, a poorly placed pulse oximeter can give a false high reading.
- Equipment Malfunction: All devices have a lifespan. A malfunctioning sensor, due to wear and tear, can lead to erroneous data.
It’s crucial to understand how these factors contribute to measurement errors. A meticulous eye on these elements ensures a cleaner line on data integrity.
Environmental Influences
The environment can wreak havoc on oxygen measurement devices. Various factors come into play:
- Temperature Fluctuations: Extreme temperatures can affect the performance of sensors, leading to skewed results. For example, a sensor that’s too hot may overstimate oxygen levels.
- Humidity Levels: High humidity can cause condensation on devices, disrupting their function, especially with optical sensors.
- Interference from Other Gases: In complex environments, the presence of gases like carbon monoxide can interfere with measurement. The sensor might mistake these gases for oxygen, leading to inflated readings.
Until measures are taken to compensate for these environmental variables, accuracy remains elusive.
"In the realm of oxygen measurements, understanding the nuances of error sources and environmental dynamics is not just optional; it’s essential."
Approaching measurement precision with a clear awareness of these challenges is fundamental. Educators, researchers, and professionals alike must be well-versed in the limitations embedded in their measurement techniques to combat these pitfalls successfully. By recognizing these hurdles, it becomes easier to employ the right strategies to mitigate their effects.
Future Directions in Oxygen Measurement
As we explore the future of oxygen measurement, it’s crucial to recognize the ongoing evolution in this field. Today, more than ever, the integration of innovative technologies into oxygen assessment techniques is central to enhancing accuracy, convenience, and overall effectiveness. The significance of this topic transcends mere academic interest; it holds profound implications for health care providers, environmental scientists, and industrial professionals alike. The pressing need for reliable and precise oxygen monitoring could not be more pertinent given the increased awareness around health, safety, and environmental sustainability.
Integration with Digital Health
The rise of digital health technologies is transforming how we monitor various physiological parameters, including oxygen levels. The integration of wearable devices that offer continuous oxygen saturation monitoring exemplifies this trend. Devices like smartwatches and fitness trackers equip individuals not only with the ability to keep tabs on their health but also to retrieve real-time data on their oxygen levels.
- Remote Monitoring: For patients with chronic respiratory conditions, having a device that tracks oxygen saturation in real-time allows healthcare providers to monitor their health remotely. This reduces the need for frequent hospital visits and empowers patients in managing their own health.
- Data Analytics: Coupling oxygen measurements with robust data analytics tools can lead to early detection of health deteriorations. By employing machine learning algorithms, healthcare systems can better predict potential health crises based on trends in oxygen data. The predictive capabilities can be a game-changer in emergency care.
- Patient Engagement: Enhanced patient engagement is also a byproduct of integrating oxygen measurement within digital health ecosystems. Users receive valuable feedback and actionable insights on their health, fostering a proactive approach to managing conditions related to oxygen levels.
Interdisciplinary Approaches
The future of oxygen measurement is not confined to singular disciplines; rather, it invites a blend of knowledge from various fields to spur advancements. A multidisciplinary approach opens doors to more holistic solutions that can cater to the intricacies of oxygen measurement.
- Collaboration Among Fields: Imagine a collaboration between biomedical engineers, data scientists, and environmental specialists. Their collaboration could lead to the development of sophisticated algorithms that take into account environmental factors while measuring oxygen, thus increasing accuracy.
- Educational and Research Institutions: Partnerships between educational institutes and industries can foster research on new sensing technologies. This can inspire innovative systems that improve current methods of measuring oxygen levels, leading to further scientific discoveries and pragmatic solutions.
- Public Health Considerations: The attention paid to interdisciplinary research could also result in public health initiatives that address issues like air quality. By measuring ambient oxygen levels alongside pollutants, public health authorities can create comprehensive strategies for improving air quality and, consequently, public health.
The interplay between different fields is where true innovation blooms. By harnessing collective expertise, we can reimagine the future of oxygen measurement not just as a task but as an essential component of health and environmental stewardship.
The End and Summary
In concluding our exploration into measuring oxygen levels, it is essential to underscore the profound significance of accuracy and reliability in these processes. Throughout the various segments of this article, we've navigated the intricate landscape of oxygen measurement methodologies, each bringing forth distinct advantages and challenges. The implications extend far beyond mere numbers; they touch on health outcomes, environmental conditions, and industrial safety.
The key findings reveal that while modern techniques such as pulse oximetry and arterial blood gas analysis stand as stalwarts in clinical settings, innovative approaches like electrochemical sensors and optical technologies are beginning to carve out their own niche. All these methods need to be evaluated with a critical eye, especially regarding their limits and the potential for errors that could compromise their efficacy. Understanding each strategy's strengths and weaknesses is crucial, particularly for practitioners and researchers in the field.
Moreover, it's not just about finding available oxygen levels; it's about grasping the context and implications of those readings. For instance, in healthcare, slight fluctuations can drastically alter a treatment plan, while in industrial settings, inaccuracies could endanger worker safety. This interplay makes the study of oxygen measurement far-reaching and essential.
Key Findings
- Diverse techniques: We have distinguished several methods for measuring oxygen, each with unique pros and cons.
- Importance of Accuracy: The reliability of these measurements is paramount, particularly in healthcare and environmental applications.
- Emerging Technologies: Newer technologies, such as nanotechnology-based sensors, promise greater precision and wider applications.
"The ability to measure oxygen levels accurately is not merely a technical requirement; it is a cornerstone of effective intervention in patient care, environmental management, and industrial processes."
Final Thoughts
In reflecting upon future directions, it’s clear that the integration of interdisciplinary approaches will likely enhance the tools and techniques used across various sectors. The need for high-quality oxygen measurement will only ascend, particularly in our shifting climate and increasing health challenges. As such, ongoing research and development in the field are pivotal. Future investments could unlock even greater precision and affordability, fundamentally altering how we interact with our environment and improve human health.
In this rapidly changing landscape, staying informed and adaptable will be key for students, researchers, educators, and professionals engaged in this important field. The dialogue surrounding the importance of accurate oxygen measurement must continue as it carries significant consequences for human life and ecological balance.