Innovative Approaches to Efficient Electricity Storage
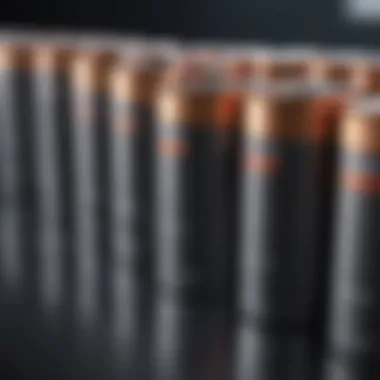
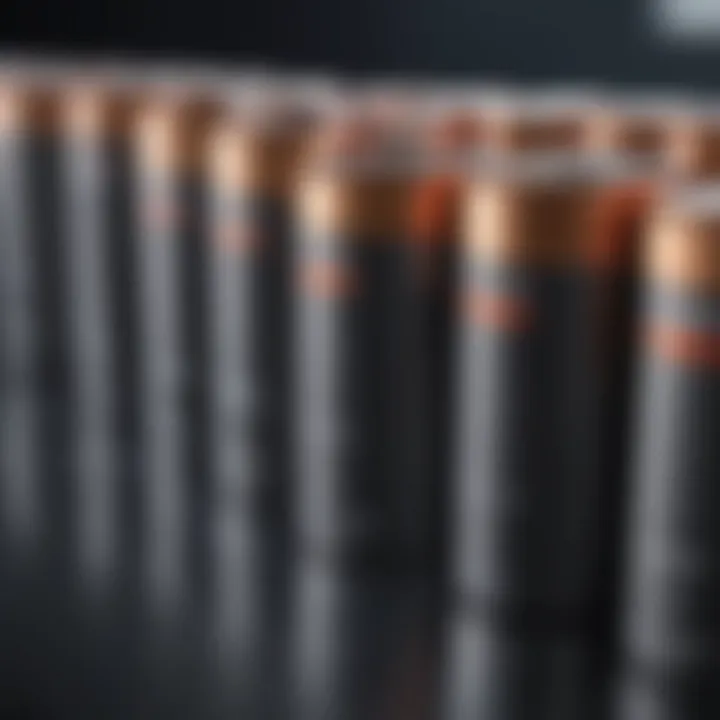
Intro
Electricity storage is an essential component in modern energy systems. As the global demand for energy rises, the need for efficient and reliable storage methods becomes increasingly critical. This article delves into various technologies that enable electricity storage, examining their efficiency, scalability, and environmental impact.
Energy storage serves multiple functions, ranging from stabilizing the grid to allowing renewable energy integration. It plays a crucial role in balancing supply and demand, especially when generation varies, such as in solar or wind power.
The advancements in storage technology are vital for achieving sustainability goals and transitioning to cleaner energy sources. By exploring contemporary methods such as batteries, pumped hydro storage, and thermal energy storage, along with innovative solutions like supercapacitors and flywheels, this article aims to provide a comprehensive guide for students, researchers, and professionals in the energy sector.
Prelude
Electricity storage presents a crucial component of modern energy systems. As reliance on intermittent renewable sources such as wind and solar grows, finding efficient methods to store electricity becomes ever more significant. These technologies not only enable energy to be stored when generated and released when needed but also enhance grid stability and support demand management.
Furthermore, the advancements in energy storage technologies contribute to a reduction in greenhouse gas emissions, aligning with global initiatives to combat climate change. With the ability to use stored energy during peak demand times, these systems help in optimizing energy consumption, thus fostering the transition towards sustainable energy practices.
Importance of Electricity Storage
The importance of electricity storage cannot be overstated. First, it addresses the mismatch between energy production and consumption. For many renewable sources, the generation peaks at times when demand is low. Electricity storage leads to a solution, ensuring that energy generated during sunny or windy periods can still be utilized later.
Second, electricity storage systems enhance grid reliability. By storing energy, these systems provide backup during outages or peak demand events. They allow for smoother transitions in energy flow, reducing the risk of blackouts.
Additionally, storage contributes to cost efficiency. By leveraging off-peak electricity rates, consumers can save money and effectively manage their energy usage. This economic advantage creates further incentives for industries and individuals to invest in storage solutions.
Historical Context
Examining the historical context of electricity storage reveals a significant evolution. Early methods included mechanical systems such as pumped hydro storage, which emerged in the early 20th century. These systems utilized gravitational energy, paving the way for future technologies.
In the latter part of the century, the development of batteries accelerated, particularly with the advent of lead-acid batteries, which became a standard for energy storage in vehicles and off-grid systems. However, limitations such as lifecycle and energy density necessitated ongoing research and innovation.
In recent decades, technological advancements in lithium-ion batteries marked a turning point. Their rise addressed efficiency and performance issues and opened new pathways for energy storage applications. Overall, understanding the historical development of these technologies provides insight into current trends and future potential in electricity storage.
Overview of Energy Storage Technologies
Energy storage technologies play a crucial role in our modern energy infrastructure, allowing for efficient use of electricity generated from various sources. The integration of renewable energies such as solar and wind has increased the necessity for effective storage solutions. These technologies enable us to balance supply and demand, ensuring a stable and reliable power grid. Understanding the various energy storage methods can guide investments and improvements in energy systems, making it an essential topic for students, researchers, and professionals.
Types of Energy Storage
Energy storage can be categorized mainly into mechanical, electrical, thermal, and chemical methods. Different types have unique characteristics and serve distinct purposes:
- Mechanical Storage: This includes methods like pumped hydro and flywheel storage. Mechanical systems store energy in moving parts, relying on kinetic or potential energy.
- Electrical Storage: This primarily involves batteries, specifically technologies like lithium-ion and flow batteries. These systems store and release energy as electrical power.
- Thermal Storage: Thermal energy storage stores heat or cold for later use. Common methods involve molten salt or chilled water, mainly used to enhance the efficiency of renewable systems.
- Chemical Storage: This is represented by energy stored in fuels such as hydrogen. It can be generated through water electrolysis and later converted back to electricity when needed.
Each type presents its advantages and challenges, depending on the application and requirements.
Comparative Efficiency of Methods
Assessing the efficiency of various energy storage methods is critical for optimizing performance and reducing costs. Here are some important points to consider:
- Lithium-Ion Batteries: These batteries are widely used due to their high energy density and efficiency ratings around 95%. However, their high costs and environmental concerns regarding material sourcing are notable drawbacks.
- Pumped Hydro Storage: This method has been in use for decades. With a typical efficiency around 70-90%, it remains popular for large-scale energy storage. Nevertheless, geographical and environmental constraints can limit site availability.
- Flywheel Storage: With efficiency rates nearing 90%, flywheels provide rapid response times and long cycle lives. They are ideal for short-term storage but have limitations in energy capacity compared to batteries or pumped systems.
- Thermal Storage: Depending on the technology used, thermal systems can achieve efficiencies of approximately 80-90%. Their applicability in integrating with solar or geothermal systems can enhance the overall efficiency of renewable energy strategies.
Evaluating these methods helps users select the right technology based on the specific needs and constraints of their energy projects.
Understanding the advantages and disadvantages of each energy storage method aids in designing effective energy strategies for the future.
Batteries
Batteries play a crucial role in electricity storage. They provide a reliable way to store and release energy based on demand. This quality makes them particularly relevant in the context of renewable energy sources, such as solar and wind, that produce electricity intermittently. By utilizing batteries, energy can be stored during peak production times and then used when needed, enhancing overall energy efficiency.
Lithium-Ion Batteries
Advantages
Lithium-Ion batteries are one of the most widely used types of batteries today. Their high energy density is a key characteristic, allowing them to store more energy in a smaller size compared to other battery technologies. This makes them a popular choice for portable electronics and electric vehicles, where space and weight are significant considerations. Furthermore, they exhibit a low self-discharge rate, meaning they retain their charge for longer periods.
This unique feature not only contributes to improved user convenience but also supports applications in larger-scale energy storage, such as grid energy storage. However, the high initial cost of lithium-ion batteries can be a disadvantage, particularly for large-scale deployment.
Limitations
Despite their advantages, Lithium-Ion batteries come with limitations. One significant drawback is their sensitivity to temperature extremes. Performance can degrade in very high or low temperatures, affecting their efficiency. Additionally, the materials used in these batteries, such as cobalt and lithium, raise concerns about sourcing and environmental impact. These factors play a role in their overall viability as a long-term solution for widespread electricity storage.
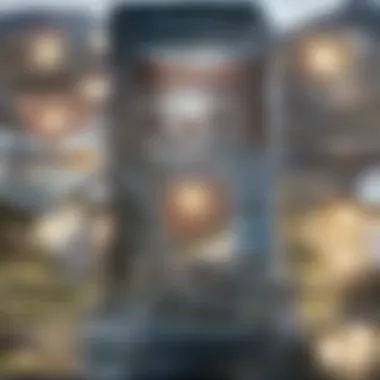
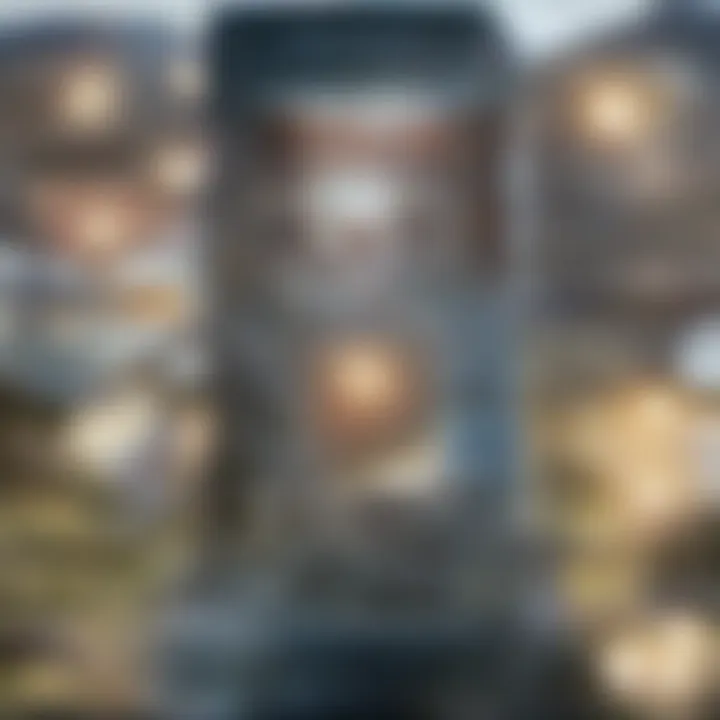
Applications
The applications of Lithium-Ion batteries are diverse. They are used in consumer electronics, electric vehicles, and increasingly in renewable energy storage systems. Their ability to support high power outputs makes them suitable for applications requiring quick bursts of energy. However, as the market for energy storage expands, it is essential to consider alternative technologies to address the limitations associated with Lithium-Ion batteries.
Flow Batteries
Mechanism
Flow batteries operate on a different principle than traditional batteries. They consist of two tanks filled with liquid electrolytes that flow through a cell where chemical reactions occur to produce electricity. This mechanism allows for scaling capacity by simply increasing the size of the tanks, making them a flexible option for larger energy storage needs. The ability to independently scale power and energy makes flow batteries particularly beneficial for renewable energy integration.
Performance
In terms of performance, flow batteries offer longer cycling lifetimes compared to Lithium-Ion batteries. They can provide a stable discharge over extended periods without significant degradation. Their ability to maintain efficiency over many charge-discharge cycles contributes to their attractiveness for grid applications. Still, they usually have lower energy density than Lithium-Ion options, which can limit their use in space-constrained applications.
Future Potential
Looking forward, the future potential for flow batteries is promising. Innovations in electrolyte materials and cell designs continue to improve their efficiency and reduce costs. Flow batteries may play a significant role in achieving higher levels of renewable energy integration as the demand for energy storage solutions grows. However, broader adoption will require continued advancements to make them more competitive with other technologies in terms of cost and energy density.
Pumped Hydro Storage
Pumped hydro storage is a vital component of energy storage solutions, particularly in large-scale scenarios. It operates by using excess electricity to pump water from a lower reservoir to an upper one. When energy demand increases, the stored water is released back down, driving turbines that generate electricity. This method is important because it provides a way to store massive amounts of energy, shifting supply to meet demand efficiently. Additionally, it plays a significant role in stabilizing the grid, especially in systems with high penetrations of renewable energy sources.
Operational Principles
The operational principles of pumped hydro storage are straightforward and depend largely on gravitational potential energy. This system usually involves two reservoirs situated at different elevations. The process can be divided into two main phases:
- Pumping Phase: When energy generation exceeds demand (often during the night or when renewables produce excess energy), the system uses that surplus electricity to pump water from the lower reservoir to the upper one. This phase typically operates at a high efficiency, around 70% to 90% depending on the specific technology.
- Generation Phase: In peak demand situations, the stored water is released from the upper reservoir back to the lower reservoir. As it flows down through the turbines, it generates electricity, which can then be fed into the power grid. The round-trip efficiency can vary but generally remains a well-performing option among energy storage technologies.
This method’s ability to respond quickly to power demands makes it a reliable means of stabilizing the electrical grid. The flow of water and the resulting conversion of kinetic energy directly into electrical energy illustrate how mechanical systems can effectively contribute to energy solutions.
Advantages and Challenges
Pumped hydro storage carries a number of advantages that underscore its importance in energy systems:
- Large Capacity: It can store vast amounts of energy, often measured in gigawatt-hours, making it suitable for large-scale applications.
- Long Lifespan: With proper maintenance, facilities can operate for decades, offering a long-term solution to energy management.
- Cost-Effectiveness: Compared to other storage options, the cost per megawatt-hour is generally lower, especially when considering the efficiency of energy conversion.
- Low Operating Costs: Once constructed, the ongoing costs of running a pumped hydro facility are relatively low, largely limited to maintenance and labor.
However, the challenges associated with pumped hydro storage cannot be overlooked:
- Geographic Requirements: Suitable sites must have specific geographical terrains, limiting where new facilities can be effectively built.
- Environmental Impact: The construction of reservoirs can disrupt local ecosystems and communities, raising environmental and social concerns.
- Initial Investment: Although operational costs are low, the initial capital required for construction can be substantial, which can deter investment.
In summary, while pumped hydro storage provides significant benefits in energy capacity and efficiency, it also comes with challenges that need careful consideration. Its operational principles and integration into the energy grid highlight its role in balancing supply and demand, critical for the future of energy systems.
Thermal Energy Storage
Thermal energy storage (TES) is crucial in the conversation about electricity storage. This method harnesses heat energy for later use, acting as a bridge between energy generation and consumption. As we shift towards renewable sources, it becomes increasingly important to find ways to balance supply and demand. TES systems have different advantages. They can store large amounts of energy at low costs, making them suitable for various applications.
One key element of thermal energy storage is its versatility. It can be used in conjunction with different renewable energy sources, like solar and wind. These systems can help reduce energy waste and increase reliability, addressing some challenges faced in energy management. The ability to store energy in the form of heat can also lead to reduced greenhouse gas emissions, contributing to environmental sustainability.
Methods of Thermal Storage
Thermal energy storage techniques can be categorized into several types. Below are common methods employed in this domain:
- Sensible Heat Storage: This method involves heating or cooling a storage medium, such as water or rocks, and using their thermal mass to store energy. For instance, water tanks can hold heated water for later use in heating systems.
- Latent Heat Storage: This technique utilizes phase change materials (PCMs) that absorb or release heat as they change from solid to liquid or vice versa. PCMs can be effective in managing temperature fluctuations in buildings, improving energy efficiency.
- Thermochemical Storage: In this method, chemical reactions are used to store energy, which is released when the reaction is reversed. It presents high energy density and allows for long-term storage, making it an attractive option for future applications.
Each of these methods has unique advantages and challenges, making them suitable for different scenarios, and they all play a role in improving the efficiency of energy systems.
Applications in Renewable Energy
Thermal energy storage has vital applications in renewable energy sectors. By integrating TES with solar power, one can store excess solar heat for use when sunlight is not available. This capability enhances solar energy's reliability and allows for an uninterrupted power supply, ultimately increasing solar's overall efficiency.
In wind energy applications, TES can help in balancing the intermittent nature of wind. By storing excess energy generated during high wind periods, it can be released when wind speeds are low, stabilizing energy supply.
Additionally, TES systems can enhance district heating systems by storing heat generated from renewable sources, thus optimizing energy use throughout the day. This capability is particularly valuable in colder regions, where energy demands peak during winter months.
"Thermal energy storage represents a significant opportunity to enhance the efficiency of renewable energy systems, making them more accessible and sustainable."
Emerging Technologies
Emerging technologies in electricity storage represent a crucial area of focus in the quest for efficient energy solutions. These technologies often provide innovative approaches that address shortcomings in existing methods, contribute to energy efficiency, and facilitate the integration of renewable energy sources. As the demand for reliable energy storage increases, emerging technologies offer unique benefits that can enhance the performance and sustainability of electricity systems.
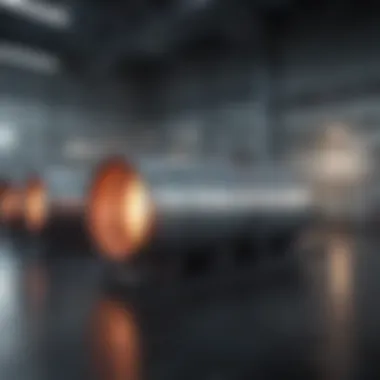
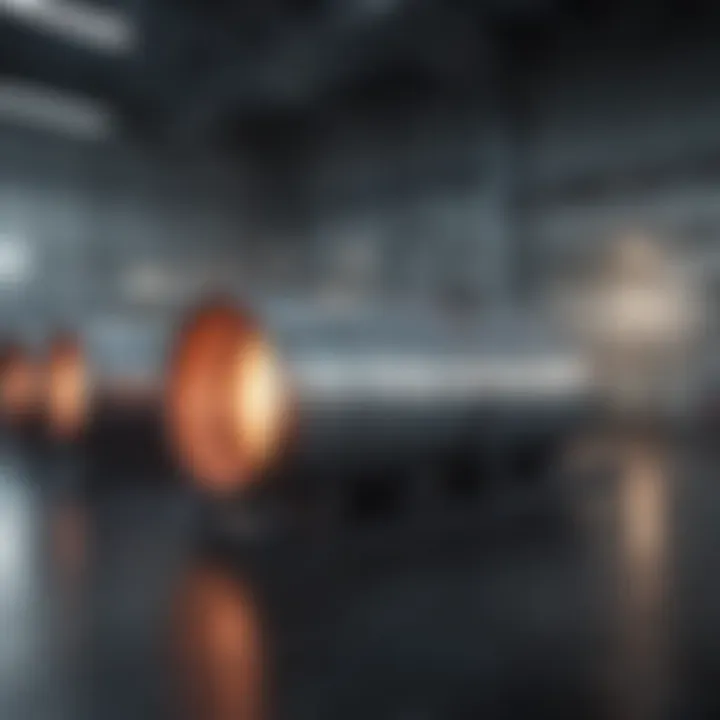
One significant factor in the discussion of emerging technologies is the pace at which they develop. Supercapacitors and flywheel energy storage systems are two examples that showcase how innovation can lead to improved storage solutions. Both of these methods stand out due to their efficiency and ability to respond rapidly to changes in energy demand. The exploration of these technologies not only emphasizes their versatility but also their potential impacts on the broader energy landscape.
Supercapacitors
Efficiency
The efficiency of supercapacitors is a defining characteristic that makes them an interesting subject in energy storage discussions. Supercapacitors have the ability to charge and discharge energy much faster than traditional batteries. This rapid response time supports applications where quick bursts of energy are necessary.
In the realm of overall energy storage, supercapacitors offer a high number of charge/discharge cycles without significant degradation. The efficiency of supercapacitors simplifies the integration of renewable energy sources, especially in situations where maintaining a stable energy supply is critical. However, the energy density of supercapacitors is lower compared to lithium-ion batteries, which may limit their applicability in certain scenarios.
Use Case Applications
The use case applications for supercapacitors are vast and varied. They find utility in electric vehicles, where quick bursts of energy enhance performance. In power grid applications, they help stabilize voltage fluctuations, ensuring reliability.
The unique feature of supercapacitors is their ability to store and release energy repeatedly without a decrease in performance. This durability and ability to handle rapid demands make them popular for various uses. However, their lower energy density means that they may not entirely replace traditional batteries in all applications. Supercapacitors are best used in conjunction with other storage systems to maximize efficiency.
Flywheel Energy Storage
Mechanics
Flywheel energy storage systems operate based on the principles of rotational energy. A flywheel consists of a rotor that spins at high speeds, storing kinetic energy. When energy is needed, the flywheel's rotation is slowed down, converting the stored kinetic energy back to electrical energy.
The mechanics of flywheel systems offer high efficiency levels, often exceeding 90%. This efficiency arises from minimal energy loss during operation. Flywheels can engage and disengage rapidly, making them well-suited for applications that require immediate energy delivery, such as grid stabilization and uninterruptible power supply systems.
Benefits and Drawbacks
The benefits of flywheel energy storage systems are numerous. They provide fast response times for energy release, making them excellent for handling sudden increases in energy demand. They also have a long operational life, typically lasting decades with minimal maintenance. Moreover, they do not employ harmful chemicals, leading to a lower environmental impact.
On the other hand, some drawbacks exist. Flywheel systems can be expensive to install and may require significant space due to the infrastructure needed for their operation. Additionally, while they are efficient, their energy storage capacity may not match that of chemical batteries, limiting their usage in certain grid applications.
In summary, both supercapacitors and flywheel energy storage systems offer promising contributions to the evolving landscape of electricity storage. Their unique features and strengths make them valuable complements in addressing modern energy demands.
Economic Considerations
The economic aspects of electricity storage play a critical role in determining the viability, adoption, and overall effectiveness of energy storage technologies. In this section, the goal is to unpack the economic factors that influence the success of different storage methods. Understanding these elements aids in evaluating options for efficient energy storage, which is increasingly necessary in a world where renewable energy sources are becoming dominant.
Effective electricity storage solutions must balance initial capital expenditures against long-term operational savings. The cost of implementing an energy storage system is a primary concern for investors and policymakers. In this context, understanding the total cost of ownership, which includes installation, maintenance, and operational costs, becomes crucial. Price competitiveness of technology also plays a large role in which storage methods are favored in different scenarios.
Perhaps the most significant aspect is the cost-benefit analysis, as it determines whether the benefits of a storage method outweigh its expenses. This analysis directly influences decision-making for investors. Financial incentives further encourage investment and adoption of specific storage systems.
Cost-Benefit Analysis of Storage Methods
A cost-benefit analysis provides a structured approach to assess the economic feasibility of various storage methods. This analysis includes a comparison of both tangible and intangible benefits against corresponding costs. Several key factors must be considered:
- Initial Costs: The upfront expenses associated with purchasing and installing the storage system.
- Operational Costs: Recurring expenses such as maintenance and energy losses during conversion.
- Revenue Generation: Potential income from energy arbitrage, selling stored energy back to the grid during peak demand, or providing ancillary services.
- Environmental Impact: While traditionally not monetized, the implications of storage technologies on emissions and ecological systems can also factor into decision-making in an increasingly eco-conscious market.
Aspects like battery degradation rates and the system's lifespan are critical to evaluating long-term benefits. For instance, lithium-ion batteries may exhibit lower initial costs but can degrade faster, leading to higher replacement frequencies.
"Understanding the economic impact of storage methods not only aids in the choice of technology but also guides policy formation."
Financial Incentives for Energy Storage Investment
Financial incentives are pivotal in promoting the adoption of energy storage technologies. Governments and private sectors can leverage various mechanisms to stimulate growth in this area. The following are common types of incentives:
- Tax Credits: Reductions in taxable income based on investments in storage systems.
- Grants and Subsidies: Direct financial assistance to offset costs of installation and operation.
- Loan Programs: Special financing options with reduced interest rates designed to lower the barrier for entry.
- Renewable Energy Certificates: Tradable commodities that represent the environmental benefits of generating clean energy, which can financially bolster storage systems.
In certain regions, enhanced regulatory policies promote energy storage integration with renewable projects. This can make storage technologies more attractive by ensuring a stable return on investment, thereby supporting energy transition goals.
Through examining economic considerations carefully, stakeholders can make informed decisions that promote not only the growth of energy storage but also more sustainable energy practices in the long term.
Regulatory and Policy Framework
The role of regulatory and policy frameworks in electricity storage cannot be overstated. They shape how energy storage systems are developed, funded, and integrated into the energy grid. Strong regulations and favorable policies can drive innovation, enhance market access, and facilitate sustainable growth of the energy storage sector. Conversely, a lack of clarity or supportive policies can hinder progress, leading to underinvestment in critical technologies.
Current Regulations Impacting Energy Storage
Various regulations impact energy storage technologies, influencing everything from installation to operational efficiency. These rules can vary greatly by region and often involve the following elements:
- Grid Interconnection Standards: Many regions require energy storage systems to meet specific interconnection standards. This is crucial for ensuring that devices operate safely and efficiently within the grid.
- Incentives and Subsidies: Some governments provide financial incentives, such as tax credits or grants, for the development of energy storage solutions. These incentives can significantly lower the cost of adoption, making advanced technologies accessible to a wider audience.
- Environmental Regulations: Energy storage technologies must comply with environmental standards. This is especially relevant for systems that may use hazardous materials. Regulations can dictate how these materials are used and disposed of.
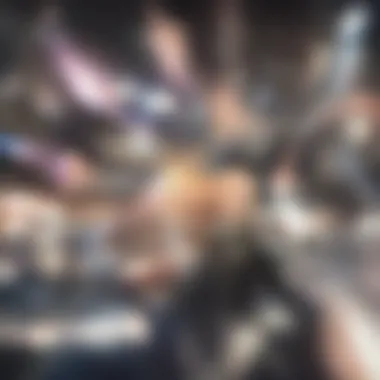
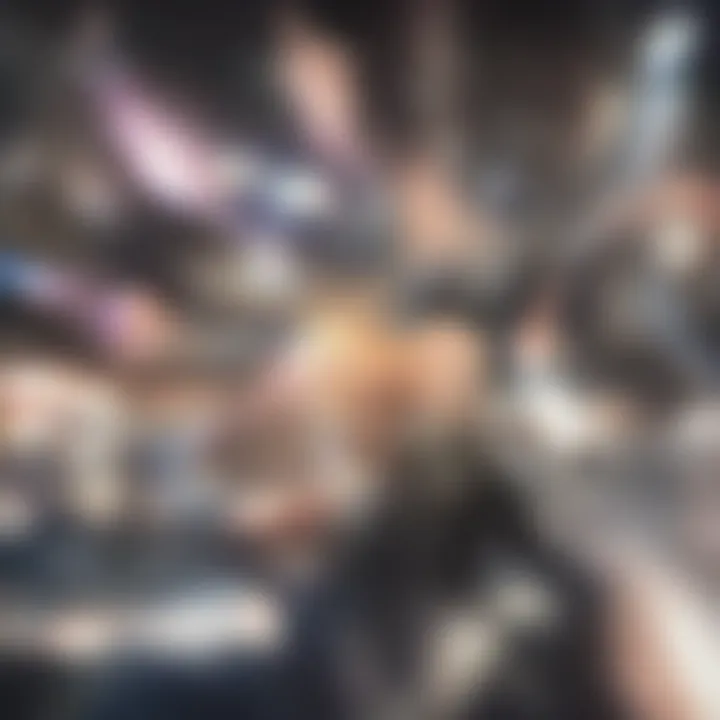
The regulatory landscape is constantly evolving, and understanding these regulations is key for innovators and investors. As the market for energy storage grows, these regulations will likely adapt, reflecting changing technology and societal needs.
Policy Recommendations for Future Development
To foster growth and innovation in electricity storage, specific policy recommendations can be made. These focus on making regulations more adaptable to emerging technologies and market conditions:
- Streamlining Approval Processes: Fast-tracking the approval process for new technologies can stimulate market entry for advanced storage solutions, making them more accessible and affordable.
- Encouraging Research and Development: Offering grants or subsidies specifically for R&D in energy storage can lead to breakthrough technologies that enhance efficiency and sustainability.
- Creating a National Energy Storage Strategy: A cohesive strategy that includes energy storage can help identify best practices, establish clear goals, and align stakeholders across sectors.
"Effective regulatory frameworks are essential for the advancement of energy storage technologies and their integration into a sustainable energy future."
Implementing these recommendations can pave the way for innovations and ultimately lead to a more resilient and sustainable energy landscape. With appropriate regulations and policies in place, the full potential of electricity storage can be realized.
Environmental Implications
Understanding the environmental implications of electricity storage has become increasingly vital in recent years as concerns over climate change and sustainability grow. With the shift towards renewable energy sources, storage solutions play a key role in balancing supply and demand. Evaluating the environmental impacts of these technologies can guide decision-making toward more sustainable practices. This section addresses the life cycle assessment of storage technologies and their long-term sustainability.
Life Cycle Assessment of Storage Technologies
Life Cycle Assessment (LCA) is a comprehensive method for evaluating the environmental impacts of a product throughout its entire life cycle. In the context of electricity storage technologies, this encompasses several stages including extraction of raw materials, manufacturing, operation, and end-of-life disposal or recycling.
- Raw Materials: The extraction phase often raises concerns. For instance, lithium mining for lithium-ion batteries can lead to water depletion and pollution. Understanding where materials are sourced from can inform policies that prioritize sustainable mining practices.
- Manufacturing Process: Production processes also affect carbon footprints significantly. Factories that produce energy storage systems may emit greenhouse gases. Efforts to decarbonize manufacturing through cleaner technologies and renewable energy sources are essential.
- Operational Efficiency: How energy storage systems operate can vary widely in their environmental impacts. For example, pumped hydro storage typically requires large reservoirs that can disrupt local ecosystems. It is important to assess such impacts during operational phases.
- End-of-Life: Finally, disposal of these products poses a challenge. Not all storage technologies are easily recyclable, which can lead to hazardous waste issues. Strategies for recycling batteries or repurposing materials must be developed to minimize landfill contributions.
Aspects of LCA can lead to better designs for sustainability, pushing innovations that are less harmful to the environment while providing substantial energy storage solutions.
Sustainability in Energy Storage
Sustainability in energy storage is about ensuring that storage methods contribute positively to the ecosystem while meeting energy demands. Several factors determine the sustainability of these technologies:
- Renewable Energy Integration: Effective storage solutions should enhance the use of renewable energy. By storing excess energy generated from wind and solar, these technologies can mitigate the intermittent nature of these sources.
- Resource Management: Emphasizing the use of abundant and non-toxic materials for building storage systems is crucial. Companies are increasingly looking for alternatives to rare materials, aiming to minimize environmental disruption during resource extraction.
- Efficiency: Higher efficiency rates lead to less energy loss and, subsequently, lower environmental impact. Storage systems should aim to maximize energy retention and minimize degradation to achieve sustainability.
- Community Impact: Understanding how energy storage technologies affect local communities can guide better practices. Engaging communities in planning can lead to more accepted and beneficial implementations.
"Sustainability is not a mere buzzword but a necessary framework for advancing energy storage technologies that benefit both the economy and the planet."
Future Directions in Electricity Storage
The evolving landscape of electricity storage presents opportunities and challenges that warrant careful examination. As the global demand for energy storage rises, the future directions in this field can greatly influence efficiency, reliability, and sustainability. Understanding these directions is crucial, as they hold the key to improving energy systems and addressing climate change.
One significant element in future electricity storage is advancements in battery technology. New chemistries, such as solid-state batteries, promise higher energy densities and enhanced safety compared to current lithium-ion solutions. Emerging technologies, such as lithium-sulfur and sodium-ion batteries, are being researched for potential widespread application. The benefits of these developments would not only include more efficient storage but could also lower costs, making energy storage accessible to diverse markets.
Considerations about renewable integration further emphasize the importance of looking toward future directions. As more renewable sources like solar and wind become prevalent, the role of storage in balancing these intermittent resources becomes critical. The ability to store excess energy during peak production hours to be used during low production hours can ensure a more stable and reliable energy supply.
"Innovative research is essential for advancing energy storage solutions and achieving sustainability goals."
Innovative Research and Development Trends
The field of electricity storage is witnessing a surge in innovative research and development. Researchers are actively exploring materials and processes that can revolutionize energy storage capabilities. For example, organic redox-flow batteries utilize environmental-friendly materials, offering a sustainable alternative to conventional batteries. This innovative approach could help reduce toxic waste associated with battery production and disposal.
Another notable trend is the development of advanced energy management systems. These systems utilize artificial intelligence (AI) and machine learning to optimize energy storage and distribution processes. By analyzing real-time data, these technologies can help enhance efficiency and reduce operational costs.
Additionally, research is focused on enhancing the lifecycle of storage technologies. Efforts to develop recycling methods for spent batteries are critical. As the demand for batteries increases, the need for sustainable disposal and reintegration of materials into the production cycle becomes vital.
Potential Market Evolution
As technological advancements continue, the market for electricity storage is anticipated to evolve significantly. The rise of electric vehicles and renewable energy systems is driving demand for efficient storage solutions. Market analysts project substantial growth in the energy storage sector as more industries recognize the need for reliable power sources.
Factors such as government incentives and policies also play a key role in shaping market trends. For instance, subsidies for energy storage systems could spur widespread adoption. Additionally, improvements in technology may lower costs, making energy storage accessible to individual consumers and businesses alike.
In summary, the future directions in electricity storage are characterized by innovative research, advancements in technology, and changes in market dynamics. These factors collectively contribute to the potential for enhanced efficiency and sustainability in energy systems, which is vital for meeting the energy demands of tomorrow.
Finale
In this article, the significance of electricity storage has been thoroughly examined. As energy needs expand globally, efficient storage solutions are paramount. They create balance between energy generation and consumption. Notably, advancements in technology offer various pathways for improvement.
Summary of Key Insights
The exploration of different energy storage methods reveals essential insights. Each technology, whether it be lithium-ion batteries, pumped hydro, or thermal storage, presents unique benefits and limitations. Key takeaways include:
- Lithium-Ion Batteries: High energy density and widespread applications, but limited lifespan and resource dependency.
- Pumped Hydro Storage: Proven technology with large-scale benefits, though geography limits where it can be applied.
- Thermal Energy Storage: Offers cost-effective options for integrating renewable sources.
- Emerging Technologies: Supercapacitors and flywheels show promise for faster charge and discharge cycles.
Understanding these technologies is crucial for making informed decisions on investment and policy. Each method contributes significantly to creating a sustainable energy future, supporting the necessity of ongoing research and development.
Final Thoughts on the Future of Electricity Storage
Looking ahead, the future of electricity storage is shaped by several factors. Innovation in battery technology is essential. Scientists are exploring solid-state batteries, which promise to enhance safety and performance significantly.
Moreover, policy advancements and financial incentives can accelerate the adoption of energy storage systems. As regulations evolve, it may lead to greater integration of energy storage into existing infrastructures.
The collaboration between public and private sectors is vital to unlocking new potentials. By investing in efficient storage solutions, we can create a resilient energy landscape ready for the challenges of tomorrow.
The future of energy storage is not just about technology. It's about our commitment to sustainability and innovation.