Expression Plasmids: Key Mechanisms in Biotechnology
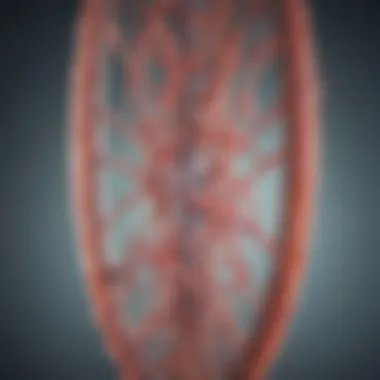
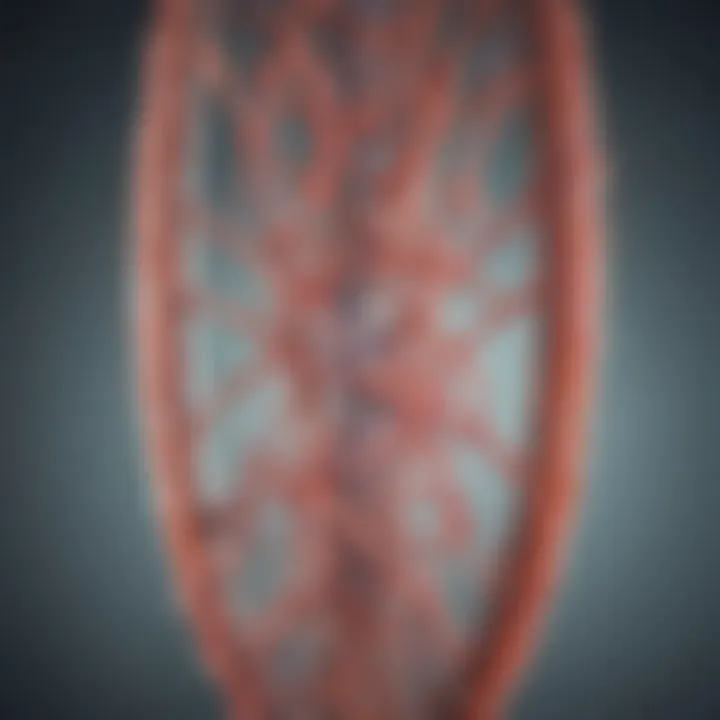
Intro
Expression plasmids are at the heart of biotechnology, serving crucial roles in gene expression and protein synthesis. With the rise of genetic engineering and molecular biology, the importance of understanding how these plasmids function has increased tremendously. Their adaptability and manipulation make them indispensable for both research and industrial applications. In this article, we will take a closer look at the multifaceted nature of expression plasmids and examine how they contribute to advancements in various sectors.
Research Context
Background and Rationale
To appreciate the role expression plasmids play, it's imperative to grasp the historical context and scientific relevance surrounding their development. Expression plasmids are essentially circular DNA molecules that are used to introduce specific genes into host cells. This process allows for the production of proteins that can be used for a variety of purposes, from therapeutic applications to industrial enzymes.
"The journey of genetic engineering began decades ago, paving the way for the sophistication we see today."
Research on expression plasmids emerged alongside the discoveries of recombinant DNA technology in the 1970s. Scientists experimented with manipulating genetic material, laying the groundwork for what would later evolve into biotechnology as we know it. Today, these plasmids support vital research in fields such as medicine, agriculture, and environmental science.
Literature Review
Over the years, extensive studies have been conducted to explore the capabilities and limitations of expression plasmids. Research articles and journals have documented the nuances of plasmid design, coding sequence optimization, and host cell selection. Key findings often highlight factors such as:
- Plasmid Structure: The arrangement of promoters, ribosome binding sites, and terminators affects protein yield.
- Cloning Techniques: Methods like restriction enzyme digestion and ligation play a pivotal role in plasmid construction.
- Host Organisms: The choice of bacterial or eukaryotic cells can impact the expression levels and post-translational modifications of proteins.
These insights help researchers enhance the efficiency of plasmid use, making their applications even more versatile. Despite advancements, challenges still remain, prompting ongoing investigations into optimizing expression systems further.
Methodology
Research Design
The approach to studying expression plasmids often employs a combination of experimental and analytical methods. Researchers may start with the design and construction of novel plasmids, followed by transfection or transformation into host cells. This systematic experimentation allows for tailored plasmid development for specific intended outcomes.
Data Collection Methods
Data collection in the realm of plasmid research can be meticulously executed through various methods:
- Biochemical Assays: These tests determine the activity and stability of proteins produced by the plasmids.
- Sequencing Techniques: Gene sequences are analyzed to confirm successful cloning and expression.
- Protein Purification: Techniques like affinity chromatography may be utilized to isolate target proteins for further study.
All these methods serve a singular purpose: to refine our understanding of how expression plasmids can be harnessed effectively in both laboratory settings and larger-scale applications. This groundwork leads to a profound mastery of techniques that benefits both scientific knowledge and practical solutions.
Prelims to Expression Plasmids
In the realm of biotechnology, expression plasmids stand as vital players. They are specifically engineered to facilitate the expression of genes, ultimately translating genetic blueprints into proteins essential for various applications. Their significance lies not just in the production of proteins but in how they enable a deeper understanding of biological processes and pave the way for medical advancements.
Definition and Importance
Expression plasmids are circular DNA molecules used as vectors to introduce and amplify foreign DNA within a host cell. They often contain essential elements that drive gene expression, such as promoters, selectable markers, and multiple cloning sites. The importance of expression plasmids can be highlighted through several critical aspects:
- Protein Production: Enabling the synthesis of recombinant proteins, which have vast applications in therapeutic development and industry.
- Research Utility: Allowing scientists to study gene function and regulation, which is pivotal in understanding diseases and developing treatments.
- Biotechnological Applications: Such as manufacturing vaccines, enzymes, and other bioproducts that enhance quality of life.
Their versatility makes expression plasmids indispensable in various fields, such as molecular biology, genetic engineering, and medicine.
Historical Context
The journey of expression plasmids began several decades ago, coinciding with the birth of recombinant DNA technology. In the 1970s, scientists first successfully inserted bacterial DNA into plasmid vectors, setting the stage for cloning techniques. Initially, these methods were crude, but as knowledge of molecular biology grew, so did the sophistication of plasmid design.
The introduction of strong bacterial promoters in the 1980s revolutionized gene expression, allowing for higher yields of recombinant protein. Over the years, enhancements have included the development of mammalian expression systems and designers with streamlined cloning approaches.
The once-simple plasmid has evolved into complex tools that can target specific cellular pathways or produce specialized proteins, reflecting how technological advancements in genetics hold promise for the future. Through this historical lens, it becomes clear that expression plasmids are not just tools but instruments of discovery that continue to influence both fundamental research and applied sciences.
Key Components of Expression Plasmids
Understanding the components of expression plasmids is essential for anyone delving into the world of biotechnology. These elements govern how effectively a plasmid can facilitate gene expression and protein production. Each part plays a strategic role, contributing not only to the plasmid's function but also to its overall utility in various applications. The combination of these components ultimately influences factors like yield and stability of the expressed proteins.
Origin of Replication
The origin of replication is like the starting gun at a race; it sets everything in motion. This component is crucial as it allows the plasmid to replicate independently within the host cell. Without a compatible origin, the entire endeavor of introducing foreign genes can be in vain. A well-chosen origin of replication ensures that the plasmid can multiply when the host cell divides, allowing for sufficient quantities of the plasmid to be available for protein expression. Different plasmids utilize distinct replication origins, which can vary based on the host organism, making the selection process a pivotal consideration in design.
Promoter Elements
Promoter elements act as the command center for gene expression, dictating when and how much of the target protein is produced. These sequences are recognized by RNA polymerase, initiating transcription of the downstream gene. The strength and type of promoter can drastically influence protein yield. For instance, a strong promoter might drive high levels of expression, which is ideal for producing large quantities of protein. However, there can be a downside; if the expression is too high, it could overwhelm the host's cellular machinery, causing issues related to protein misfolding or toxicity. Balancing promoter strength is therefore a fine art, requiring careful consideration based on the goals of the experiment.
Selectable Markers
Selectable markers serve as a safeguard, allowing for the identification of successfully transformed cells. These markers, often antibiotic resistance genes, enable researchers to discern which cells have taken up the plasmid. For example, in the presence of an antibiotic, only those cells harboring the resistance gene can survive, making it relatively straightforward to isolate desired clones. However, it's important to select markers judiciously; using widely exploited markers can lead to contamination and false positives. Thus, it requires an understanding of the entire experimental context to optimize marker choice effectively.
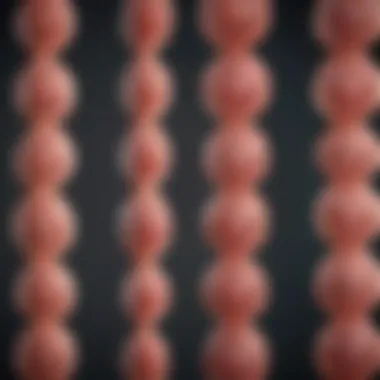
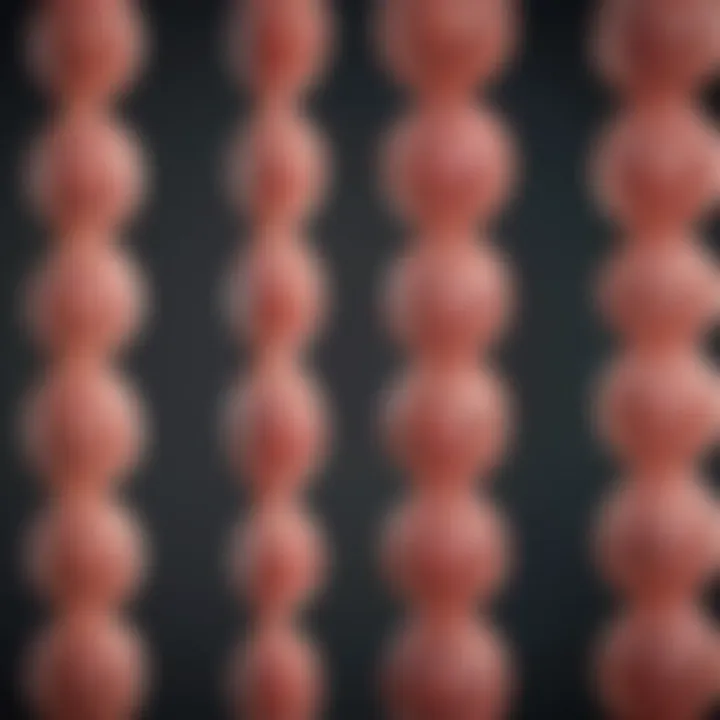
Multiple Cloning Sites
Multiple cloning sites (MCS) are like a Swiss army knife in the toolkit of molecular biologists. MCS are regions within a plasmid that contain several unique restriction sites, allowing for multiple cloning options. This flexibility is invaluable for inserting different genes into the plasmid. By facilitating various cutting and pasting methods, MCS enable scientists to adapt their cloning strategies to best suit their experimental needs. Researchers often prefer vectors with multiple cloning sites that offer a range of endonuclease options, enhancing their ability to manipulate genetic constructs effectively.
In summary, these key components contribute significantly to the effectiveness and efficiency of expression plasmids in biotechnological applications. Understanding their roles allows researchers to make informed choices in experimental design, ultimately influencing the success of their projects.
Strategies for Cloning in Expression Plasmids
Cloning strategies play a pivotal role in the manipulation of expression plasmids, essentially guiding the processes that enable researchers to insert genes of interest into plasmids. The choice of a cloning method can significantly affect the efficiency, fidelity, and ultimately the success of gene expression. To navigate the diverse landscape of cloning techniques, it is essential to understand their individual mechanisms, advantages, limitations, and practical considerations.
Restriction Enzyme Cloning
Restriction enzyme cloning is a traditional yet foundational method widely utilized in molecular biology. This technique employs specific enzymes, known as restriction endonucleases, that cut DNA at precise sequences, creating sticky or blunt ends conducive for ligation.
By using a well-planned combination of these enzymes, researchers can tailor the cut sites to accommodate their particular genes of interest, providing a customizable approach to cloning. The resulting DNA fragments can then be mixed with a suitable vector and ligated, yielding a recombinant plasmid.
Advantages of this method include:
- Precision: Restriction enzymes recognize specific sequences, allowing for predictable manipulation of DNA.
- Established Protocols: Decades of refinement have led to widely accepted methodologies, making it a reliable choice.
- Cost-Effective: Materials needed for this techniques are often less costly than newer methods.
However, this approach has its downsides. The reliance on specific restriction sites can limit flexibility, prompting researchers to redesign constructs if those sites are unavailable in the target gene.
Gibson Assembly
Gibson Assembly offers a more contemporary alternative to traditional methods, allowing for the seamless joining of multiple DNA fragments in a single reaction. This method exploits the principles of DNA homology, wherein overlapping regions between the adjacent fragments facilitate their fusion.
In practice, researchers first generate overlapping sequences by designing primers or PCR products that have overlapping ends. The fragments are then mixed with the Gibson Assembly Master Mix, which contains a combination of enzymes that facilitate the joining process:
- Exonuclease: Creates single-stranded ends to enhance annealing.
- Polymerase: Fills in gaps and completes the strands.
- Ligase: Seals the nicks, resulting in a complete plasmid.
Benefits of Gibson Assembly include:
- Versatility: Multiple fragments can be assembled simultaneously, providing greater versatility in constructing complex plasmids.
- No Restriction Sites Needed: This method bypasses the need for restriction sites, allowing for a more straightforward design.
- Efficiency: The streamlined approach can save time in the lab.
While Gibson Assembly has many advantages, it also requires careful design to ensure effective overlapping sequences. Miscalculations in the designs can result in inefficient assemblies.
Gateway Cloning Technology
Gateway Cloning Technology stands out for its efficiency, allowing for a versatile system to transfer DNA sequences between plasmids without the need for restriction enzymes. At the core of this method is the concept of recombination; researchers make use of specific site sequences called "att sites", recognized by recombinant enzymes.
The process begins by inserting the gene of interest into a donor plasmid that contains the necessary att sites. Upon mixing with a compatible destination plasmid, recombination occurs, precisely integrating the gene into the new plasmid without any extraneous sequences.
Key aspects of Gateway Cloning include:
- Simplicity: The approach simplifies the cloning process, as no ligation or restriction enzymes are necessary.
- Flexibility: It offers a high degree of flexibility in transferring genes between different vectors.
- Speed: This method can be considerably faster compared to traditional cloning approaches.
Nevertheless, there are some challenges associated with Gateway Cloning. The requirement for specific recombination sites necessitates additional design considerations, which can complicate initial planning.
"Each cloning strategy possesses unique merits and challenges that researchers must weigh carefully based on their specific project goals and resources."
Through these detailed methods—Restriction Enzyme Cloning, Gibson Assembly, and Gateway Cloning Technology—scientists can effectively utilize plasmids in expression systems, ultimately driving advancements in biotechnology and genetics. Evaluation of these strategies allows researchers to select the most appropriate one based on the complexity of the gene, the tools available, and the objectives of their study.
Expression Systems for Plasmids
Expression systems are critical in the utilization of plasmids for biotechnology. They dictate how genes inserted into plasmids are expressed within host organisms. Different systems lead to variations in protein yield, folding, and functionality. Understanding these systems helps researchers select the most suitable option for their specific project needs. Consequently, this knowledge forms the backbone of effective biotechnological applications ranging from basic research to the development of therapeutics.
Bacterial Systems
Bacterial systems, like Escherichia coli, are the workhorses of gene expression due to their simplicity and speed. When plasmids are introduced into these bacteria, they take on the tasked of producing the desired protein.
Some specific benefits include:
- Rapid Growth: Bacteria multiply quickly, yielding large amounts of product in a short timeframe.
- Cost-Effective: Cultivating bacteria is less expensive than other systems, making it feasible for larger-scale projects.
- Established Protocols: There are numerous well-understood methods for working with bacterial expression systems, making it accessible for beginners.
However, bacterial systems come with their own set of challenges. For instance, bacteria often struggle with post-translational modifications which limits the proper folding and activity of some eukaryotic proteins. Ultimately, the choice of a bacterial system hinges largely on the characteristics of the target protein and the degree of complexity required.
Yeast Systems
When researchers require a more sophisticated expression system, yeast systems, like Saccharomyces cerevisiae, are often the candidate of choice. They are eukaryotic organisms, offering better post-translational modification capabilities while still being easier to manipulate than higher organisms.
The advantages of using yeast systems include:
- Eukaryotic Features: Yeasts provide the necessary machinery for modifications such as glycosylation that many bacterial systems lack.
- Ease of Genetic Manipulation: Many protocols exist for modifying yeast genomes effectively.
- High Yields: Under optimal conditions, yeast can produce proteins at a scale comparable to bacterial systems.
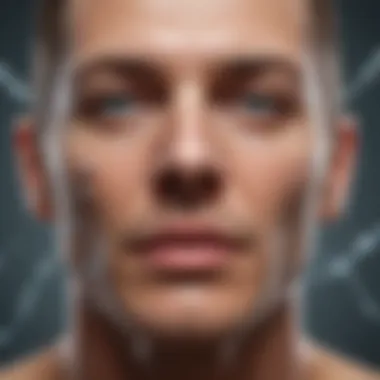
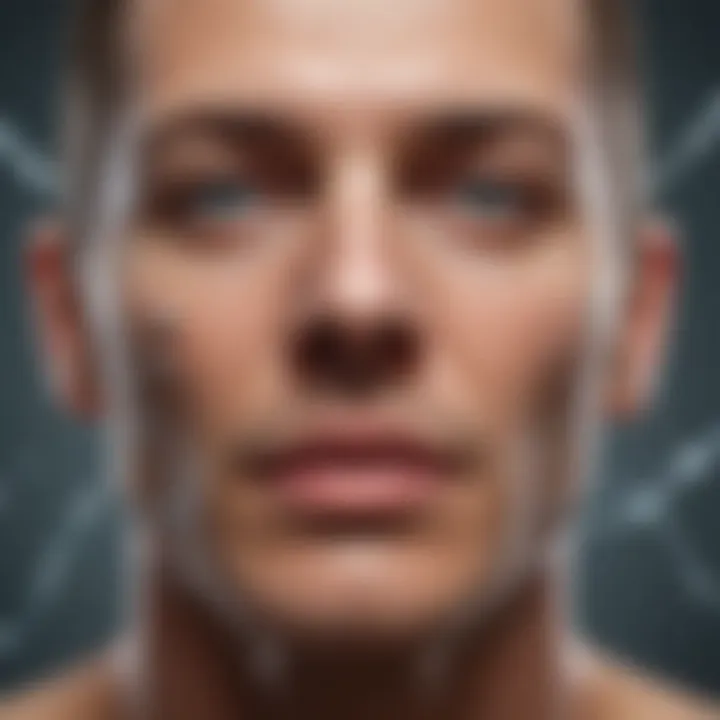
Nonetheless, they aren’t without limitation. There's a need for consistent culture conditions, and some yeast strains can be finicky, affecting protein expression levels. Additionally, the glycosylation patterns can differ from mammalian systems, which may lead to functional discrepancies in therapeutic contexts.
Mammalian Cell Lines
In cases where proteins require an environment most similar to human physiology, mammalian cell lines come into play. These systems accurately replicate the necessary cellular activities for complex protein expression and modifications.
Notable benefits of using mammalian cell lines include:
- Correct Folding and Function: These systems produce proteins that more closely resemble their natural forms, crucial for functions in therapeutic applications.
- Glycosylation Patterns: They provide the correct enzymatic environment for specific post-translational modifications that are essential for biological activity and stability of therapeutic proteins.
- Reusable Systems: Established cell lines can be maintained over time, allowing for continuous protein production.
Yet, the intricacy of working with mammalian cells is a significant hurdle. These systems are more costly and time-consuming compared to bacterial or yeast systems. The intricacies of tissue culture require skill and attention to detail, which may limit their ease of use in routine settings.
Applications of Expression Plasmids
Expression plasmids have a versatile role that extends far beyond simple gene transfer. They serve as powerful tools in various biotechnological applications, significantly impacting areas such as recombinant protein production, vaccine development, gene therapy, and functional studies of protein interactions. The use of expression plasmids adds a layer of precision and efficiency in these fields, making them indispensable in modern biotechnology. Understanding these applications is crucial for researchers and professionals to harness the full potential of plasmids in their work.
Recombinant Protein Production
Recombinant protein production is perhaps one of the most celebrated utilizations of expression plasmids. In this process, a gene coding for a protein of interest is introduced into a suitable host organism, typically bacteria, yeast, or mammalian cells, using a plasmid as the vector. This technique allows researchers to produce large quantities of proteins that may be difficult or impossible to isolate from natural sources.
The benefits are numerous. Cost-effective production methods, the ability to carry out post-translational modifications, and the high yield of target proteins make this approach highly attractive. For instance, insulin production has transitioned from extraction from animal pancreas to recombinant methods, which steadily meet the growing demands of diabetes management. Additionally, researchers can engineer specific traits into proteins, thereby enhancing functionality or stability.
In practical applications, plasmids such as pET or pGEX are widely utilized, making the process smoother than a hot knife through butter.
Vaccine Development
The role of expression plasmids in vaccine development cannot be overstated. With the surge of interest in genetic vaccines, expression plasmids allow the safe introduction of antigens into host cells, triggering an immune response without the risk of causing disease. For example, plasmid DNA vaccines for zoonotic diseases are in development, providing protection against infections while being easier to manufacture and store compared to traditional vaccines.
Moreover, the capacity for quick modifications means that when new pathogens emerge, researchers can adapt existing plasmids relatively swiftly. Take the COVID-19 vaccines, where some formulations utilized plasmid technology to prompt the production of viral proteins, training the immune system effectively and efficiently.
Gene Therapy
Gene therapy is an expansive and increasingly promising field, relying heavily on expression plasmids for the delivery of therapeutic genes directly into patients' cells. This method holds potential for treating genetic disorders caused by defective genes, such as cystic fibrosis or hemophilia.
By introducing corrected genes using plasmids, the aim is to restore normal function to the affected cells. However, several hurdles exist, such as ensuring stable integration and expression of the introduced genes. The challenges include immune responses against the plasmids themselves and stability of the gene once inside the host.
Yet, advancements continue, and expression plasmids are continually refined to enhance efficacy and safety, aiming to make these therapies mainstream.
Functional Studies of Protein Interaction
Understanding protein interactions is pivotal in molecular biology, providing insights into cellular functions and signaling pathways. Expression plasmids facilitate the study of these interactions by enabling the simultaneous expression of multiple proteins within a single cell. This application often employs tagged versions of proteins, aiding in the identification of interactions through techniques like co-immunoprecipitation.
The practicality here is that one can observe how proteins address each other in real-time, which can answer critical biological questions. For example, studying how oncogenes interact at a cellular level can pave the way for novel cancer therapies. Researchers can manipulate the expression levels and observe the consequences without such technologies, it would be like finding a needle in a haystack.
"Understanding protein interactions helps unlock the mysteries of cellular processes, unveiling new therapeutic horizons."
Overall, the applications of expression plasmids showcase their pivotal role in advancing biotechnology. From producing life-saving proteins to exploring the deepest mysteries of gene function, expression plasmids remain at the forefront of innovation in scientific research and industry. The advancements in these domains continue to reshape our understanding and treatment of various diseases, propelling the field of biotechnology into an exciting future.
Challenges and Limitations
Understanding the challenges and limitations of expression plasmids is crucial for anyone delving into biotechnology. While these plasmids are incredibly valuable, their use does come with certain hurdles that need addressing. Acknowledging these challenges helps researchers make informed decisions about experimental designs and methodologies. These limitations are not just minor inconveniences; they can greatly affect the efficiency and sustainability of biotechnological applications.
Stability Issues
Stability of expression plasmids is a key factor that influences their overall effectiveness in various systems. When plasmids are introduced into host cells, it is essential that they remain intact and functional over time. For instance, plasmid degradation can occur due to nucleases, which are enzymes that degrade nucleic acids. If the plasmid is not stable, the downstream applications may yield subpar results or simply fail altogether.
Moreover, this stability can be affected by factors such as:
- Temperature fluctuations: Extreme temperatures can lead to plasmid denaturation or degradation.
- Host cell growth conditions: Variations in pH and nutrient availability can impact plasmid replication within the cell.
- Genetic interactions: The presence of other competing genetic elements may also undermine plasmid stability.
Consequently, researchers must consider strategies for enhancing plasmid stability, such as using various vector designs or selecting more resilient host strains.
Toxicity Effects on Host Cells
Another significant challenge involves the potential toxicity effects that expression plasmids can have on host cells. When foreign genes are introduced into a cell, the resulting protein products might be detrimental to the host's metabolism. This toxicity can stifle cell growth, leading to reduced yields of the desired proteins.
An example of this would be when a highly active enzyme is expressed; the concentration of the enzyme might reach levels that overwhelm the host's protein degradation pathways. This not only causes stress to the host but can also lead to cell death. Some indicators of toxicity could include:
- Decreased growth rates: Host cells may not proliferate as expected.
- Compromised cellular functions: Cells may exhibit altered metabolic activities.
- Protein misfolding: Excessive production of proteins can lead to aggregation, further straining the cells.
Identifying and mitigating these effects requires careful monitoring and adjustment of the expression conditions. In some cases, modifying the expression level through controlled promoters can significantly reduce toxicity.
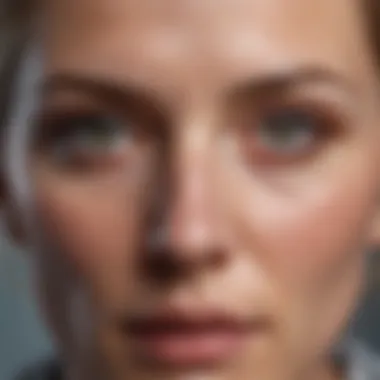
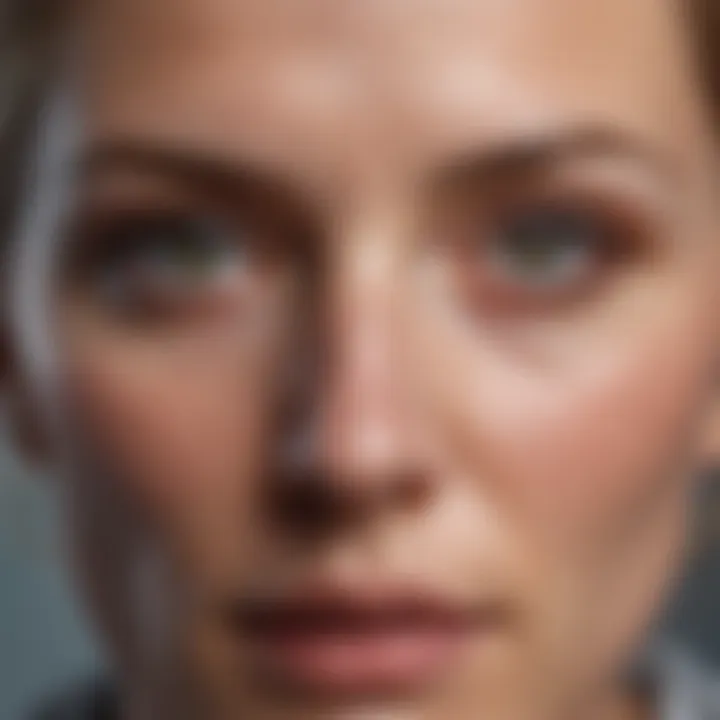
Post-translational Modifications
Post-translational modifications are another layer of complexity in the use of expression plasmids. These modifications are crucial for the functional activity of many proteins. However, not all expression systems are equipped to modify proteins in the same way that natural host systems would. Bacterial systems, for example, often lack the necessary enzymes for glycosylation, a common post-translational modification essential for the stability and activity of many eukaryotic proteins.
Key considerations in this regard are:
- Incompatibility of host systems: Certain mammalian proteins might not fold correctly in bacterial cells as they miss out on essential modifications.
- Expression levels: Overexpression of a protein can lead to incomplete or erroneous modifications, affecting protein functionality.
- Cost and time: Generating the right conditions for proper modifications can increase the complexity, time, and financial resources involved in research and development.
Recent Advancements in Expression Plasmid Technology
In the rapidly evolving field of biotechnology, expression plasmid technology has witnessed significant advancements that enhance their utility and efficiency. These innovations not only streamline genetic engineering processes but also broaden the application spectrum of plasmids in research and industrial settings. Keeping up with recent developments is crucial for students, researchers, and professionals who are navigating the complex landscape of molecular biology.
The integration of modern technologies such as CRISPR-Cas9 and synthetic biology approaches has transformed the way expression plasmids are designed and utilized. Each advancement brings specific benefits that address the traditional limitations of plasmid technology and opens new avenues for exploration.
CRISPR-Cas9 Integration
CRISPR-Cas9 has emerged as a game changer in gene editing, and its integration with expression plasmids marks a turning point in biotechnology. This approach enables targeted changes at specific sites in the genome, achieving unprecedented precision.
Using CRISPR with expression plasmids allows researchers to design plasmids that can not only express genes but also facilitate gene editing. By incorporating the Cas9 protein and guide RNA sequences directly within the plasmid, scientists can direct the expression and activity of these components specifically where needed,
- Enhanced Flexibility: This flexibility enables researchers to knock out unwanted genes or insert new ones, providing unparalleled capabilities for functional genomics.
- Reduced Delivery Methods: CRISPR plasmids can also simplify traditional delivery methods. Instead of separate systems for expression and gene editing, a single plasmid can handle multiple tasks, making it simpler and more efficient.
However, there are considerations we must keep in mind:
- Off-target Effects: Even with advanced targeting, the potential for off-target edits can create unintended consequences, requiring thorough validation.
- Complexity of Designs: The design of CRISPR expression plasmids can become intricate, potentially leading to challenges in optimization and stability.
"The integration of CRISPR-Cas9 with expression plasmids represents a frontier for precise genome editing, significantly advancing our ability to modify and understand genetic functions."
Synthetic Biology Approaches
Synthetic biology is an interdisciplinary field that fuses biology, engineering, and computer science to create complex biological systems. Recent advancements in this area have emphasized the design and construction of new biological parts, devices, and systems, including expression plasmids.
Synthetic biology approaches allow for the creation of expression plasmids with tailored properties for specific applications.
- Designer Plasmids: Researchers can now construct plasmids that contain optimized promoter regions and custom ribosome binding sites, which enhance protein yield and functionality.
- Modular Systems: This modular design facilitates easier assembly and re-configuration of plasmids depending on the application, ensuring that expression is efficient in various host organisms.
In practice, synthetic biology aims to create cells that perform complex tasks previously thought to be the domain of multicellular organisms. For example, engineered microbes can now produce biofuels or pharmaceuticals via tailor-made plasmids, which were engineered to produce specific proteins required for these processes.
Nevertheless, challenges also arise from these advancements:
- Regulatory Hurdles: The synthetic nature of these plasmids raises questions about safety and regulation within comprehensive frameworks that might not fully account for these novel constructs.
- Ethical Concerns: With great power comes great responsibility. The potential misuse of synthetic biology techniques can generate pertinent ethical discussions about bioengineering.
By embracing these advancements in expression plasmid technology, the scientific community stands on the threshold of extraordinary breakthroughs. Yet, it remains essential to approach these innovations thoughtfully, with an eye on both potential and responsibility as we continue to expand our understanding of biology.
Future Directions in Expression Plasmid Research
The field of expression plasmids is evolving rapidly, fueled by advancements in technology and a deeper understanding of molecular biology. It's not just the same old tune anymore; new possibilities are pushing the boundaries of what can be achieved. Researchers and biotechnologists need to keep their eye on the ball, as these future directions promise to reshape how plasmids are utilized in genetic research and therapeutic applications.
Enhanced Delivery Systems
The delivery of expression plasmids into target cells is often a critical bottleneck in successful gene therapy or protein production. Current methods, like electroporation or lipofection, show promise but also have limitations regarding efficiency and safety. Future directions are honing in on enhanced delivery systems, targeting issues such as biocompatibility and efficiency.
- Nanoparticles: Recent studies have explored the use of lipid-based nanoparticles for plasmid delivery. These tiny carriers can encapsulate plasmids, allowing for a more controlled and efficient release into the cells. The beauty of nanoparticles lies in their ability to overcome biological barriers that traditional methods struggle with.
- Viral Vectors: Another area ripe for exploration involves the use of engineered viral vectors. They can facilitate the introduction of plasmids with remarkable efficiency through natural cellular pathways. This approach isn't without its own set of challenges, particularly concerning safety and immune response, but with advancements in synthetic biology, these issues are gradually being addressed.
"Harnessing new delivery systems could revolutionize the field of gene therapy, making treatments more effective and accessible," recent journal articles suggest.
Targeted Gene Expression
Precision is key in modern genetics. The future of expression plasmids also lies in targeted gene expression systems that can ensure the appropriate gene only gets switched on in the right context. This specificity can reduce off-target effects and enhance the therapeutic potential of plasmids in various applications.
- CRISPR Technology: The integration of CRISPR technology is paving the way for new methodologies in plasmid design. Researchers can engineer plasmids with built-in CRISPR components to regulate gene expression with high specificity. Imagine a plasmid that knows where to go and what to do — that's becoming a reality.
- Inducible Systems: Future research is steering toward plasmids that employ inducible promoters. This means that the expression of a gene can be tightly controlled by external factors, such as certain chemicals or light. This kind of precise control can be critical in studies that require a careful balance of gene expression.
Finale
The conclusion serves as a pivotal part of this article, encapsulating the central themes and messages throughout the exploration of expression plasmids in biotechnology. As we have discussed, expression plasmids are not just mere vectors; they are indispensable tools that facilitate the intricate processes of gene expression and protein production.
Summary of Insights
In summary, this article revealed several key insights regarding expression plasmids:
- Structural Components: We highlighted the essential elements that comprise expression plasmids, such as the origin of replication, promoter elements, and selectable markers. Each component plays a critical role in enabling efficient cloning and expression in host cells.
- Cloning Strategies: We examined various cloning techniques, including traditional restriction enzyme methods and modern approaches like Gibson Assembly and Gateway cloning technology. These methods enhance the precision and efficiency of plasmid construction.
- Applications Across Fields: The versatility of expression plasmids was illustrated through various applications, from recombinant protein production to gene therapy. This underscores their impact across diverse areas, including medicine and research.
- Challenges Encountered: We also addressed the challenges that researchers face when using expression plasmids, such as stability issues and the potential toxicity to host cells. Understanding these limitations is crucial for advancing the technology.
These insights paint a comprehensive picture of how expression plasmids function within the biotechnology landscape, highlighting both their promise and pitfalls.
Implications for Future Research
Looking ahead, the implications of this research are significant. Future inquiry into expression plasmid technology holds the potential for further advancements, especially in the following areas:
- Enhanced Delivery Systems: Development of more effective delivery systems for expression plasmids could improve gene therapy outcomes, making treatments more viable and efficient.
- Targeted Gene Expression: Research can focus on optimizing plasmids for more precise gene expression control, minimizing off-target effects, and maximizing therapeutic benefits.
- Interdisciplinary Approaches: Collaborations between synthetic biology and computational modeling could yield novel plasmids tailored for specific tasks, increasing the robustness of expression systems.
- Regulatory Frameworks: As the technology evolves, establishing comprehensive regulatory frameworks will become essential for ensuring safety and efficacy in therapeutic applications.