Exploring Green Waste Energy: An Analytical Perspective
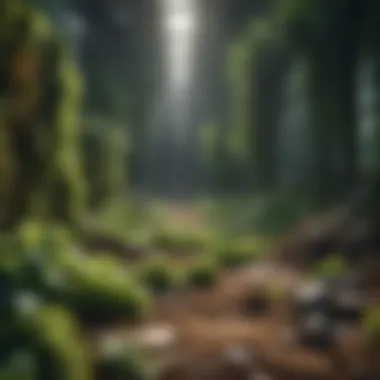
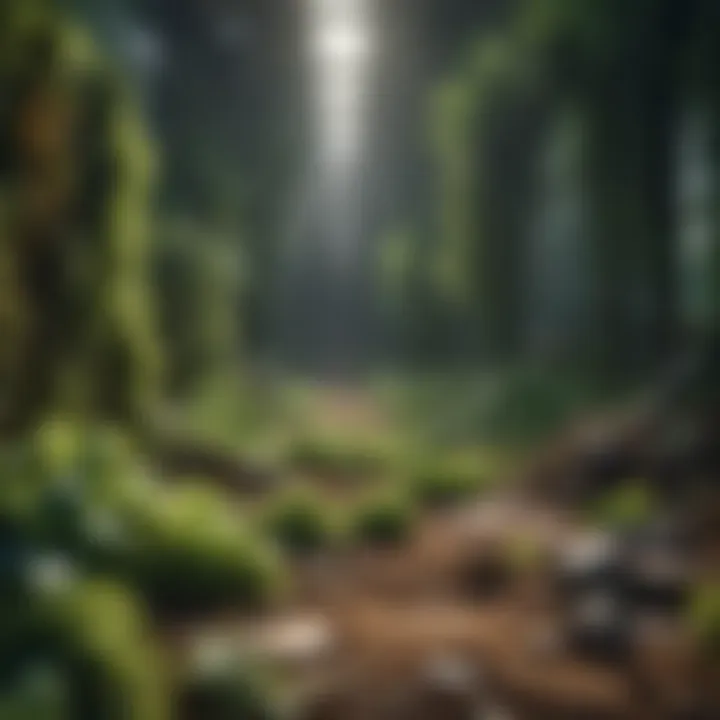
Intro
The topic of green waste energy has emerged as a significant area of interest in recent years. With the growing concern about climate change and environmental sustainability, innovative solutions are urgently needed. Green waste energy refers to the process of converting organic waste into usable forms of energy. This includes methods such as anaerobic digestion, composting, and incineration. Each method presents unique advantages and challenges, making it essential to explore this field from multiple angles.
Research Context
Background and Rationale
The rationale behind investigating green waste energy stems from various factors. First, organic waste constitutes a substantial portion of total waste generated globally. It represents a potential resource rather than merely a disposal challenge. By recycling this waste into energy, it can mitigate landfill pressure and reduce greenhouse gas emissions.
Furthermore, with the transition towards renewable energy, green waste energy plays a critical role. Understanding its mechanisms and innovation opportunities can inform policies for waste management and energy production.
Literature Review
An extensive body of research highlights various facets of green waste energy. Studies often focus on conversion techniques and their efficiency. For example, Zhao et al. (2021) examine anaerobic digestion and its role in enhancing energy recovery from food waste. Additionally, Smith and Thompson (2020) analyze the environmental impacts associated with incineration methods.
Moreover, several publications emphasize the socioeconomic implications of implementing these technologies. For instance, dense urban areas often face distinct challenges in managing green waste effectively. The literature suggests that community engagement is vital for successful adoption of these technologies.
Methodology
Research Design
This article employs a qualitative research design to explore the multifaceted aspects of green waste energy. The analysis involves synthesizing existing literature, case studies, and expert opinions to provide a well-rounded understanding of the current state of technology and its potential.
Data Collection Methods
Data for this study has been collected through various means, including:
- Literature Analysis: Review of peer-reviewed journals and articles.
- Case Studies: Examination of selected initiatives that successfully implemented green waste energy solutions.
- Expert Interviews: Consultation with professionals in the energy and waste management sectors to gain insights on current trends and future directions.
It is through these methods that a clearer picture of the role green waste energy plays in waste management and renewable energy systems can be established.
Preamble to Green Waste Energy
Green waste energy is gaining attention as a vital aspect of sustainable practices in waste management. This topic is significant for several reasons, particularly in its potential to address pressing environmental challenges such as landfill overuse and greenhouse gas emissions. With the world generating billions of tons of organic waste annually, understanding how to convert this waste into usable energy is essential. It presents an innovative solution that not only reduces waste but also contributes to the energy supply.
The benefits of green waste energy are multi-faceted. For one, it helps divert organic materials from landfills. This reduces the volume of waste that decomposes anaerobically, which is a substantial source of harmful methane emissions—a potent greenhouse gas. Furthermore, energy produced from green waste can replace fossil fuels, enhancing energy security and reducing dependence on non-renewable resources. As society becomes more inclined towards sustainability, the exploration of green waste energy is becoming increasingly relevant.
When analyzing green waste energy, it is crucial to consider the types of materials that qualify as green waste. This understanding sets the foundation for efficient conversion methods. Additionally, the processes involved in transforming biodegradable materials into energy need to be scrutinized. These methods vary in their technologies and approaches, which influences their efficiency and environmental impact.
The path to implementing green waste energy solutions does not come without challenges. Public perception and technological limitations can hinder progress. However, the potential for advancing this field and integrating it into broader renewable energy strategies is notable. Particularly, as innovations continue to emerge, the horizon for green waste energy appears promising and is worth exploring further.
Definition of Green Waste
Green waste refers to biodegradable materials primarily originating from garden, landscaping, and food waste. Typically, it includes leaves, grass clippings, branches, fruit and vegetable scraps, and even some types of paper products. These materials, when managed correctly, can be transformed into energy through various methods. In a world where organic waste constitutes a significant portion of total waste, defining green waste is the first step towards utilizing it effectively.
Overview of Waste-to-Energy Processes
Waste-to-energy processes encompass the various techniques employed to convert organic waste into energy. These processes involve both thermal and biochemical methods, each with its own advantages and limitations. Key processes include:
- Anaerobic Digestion: This involves the breakdown of organic material by microorganisms in an oxygen-free environment, producing biogas, which can be converted to electricity.
- Incineration: This is the combustion of organic waste at high temperatures, converting it into ash, flue gas, and heat energy.
- Gasification: This process involves heating organic materials to produce syngas, which can be further converted into electricity or fuels.
- Composting: While not traditionally viewed as an energy conversion method, composting can produce heat and biofertilizer, adding value to the waste.
Understanding these processes is essential for evaluating the effectiveness and sustainability of green waste energy solutions. Each method has distinct characteristics, energy yields, and implications for environmental impact.
"The potential of green waste energy is immense, serving as both a waste management solution and a valuable energy resource."
This quote underscores the importance of recognizing the dual benefits of managing waste and generating energy, which is central to this exploration.
The Science Behind Green Waste Energy
The foundation of understanding green waste energy lies in the biological and chemical principles that govern how organic materials can be transformed into usable energy. This section elucidates the specific elements involved in green waste energy, highlights the benefits provided by these processes, and addresses various considerations that must be taken into account.
Biodegradable Materials
Biodegradable materials are pivotal in the context of green waste energy. These substances comprise organic matter that can naturally decompose through microbial action. Common sources include food scraps, yard waste, and agricultural residues. Understanding the composition of biodegradable materials is essential because not all organic waste yields the same energy potential when processed.
- Composition: Different materials contain varying levels of carbon and nitrogen, which affect microbial activity during decomposition. For instance, food waste has a high-water content, making it ideal for anaerobic digestion.
- Energy Content: The energy potential of biodegradable materials can be measured through calorific value. Materials rich in lignocellulosic content, like wood, can provide higher energy outputs when processed using thermal methods.
- Waste Sorting: Effective segregation of biodegradable waste from non-biodegradable components is essential. This practice enhances the efficiency of waste management systems and improves energy recovery rates.
Anaerobic Digestion Process
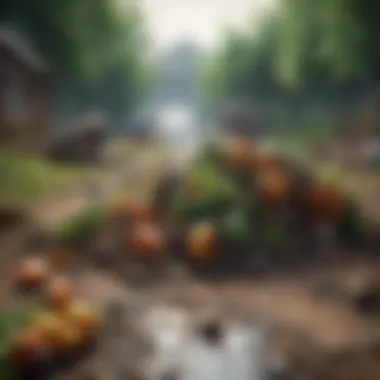
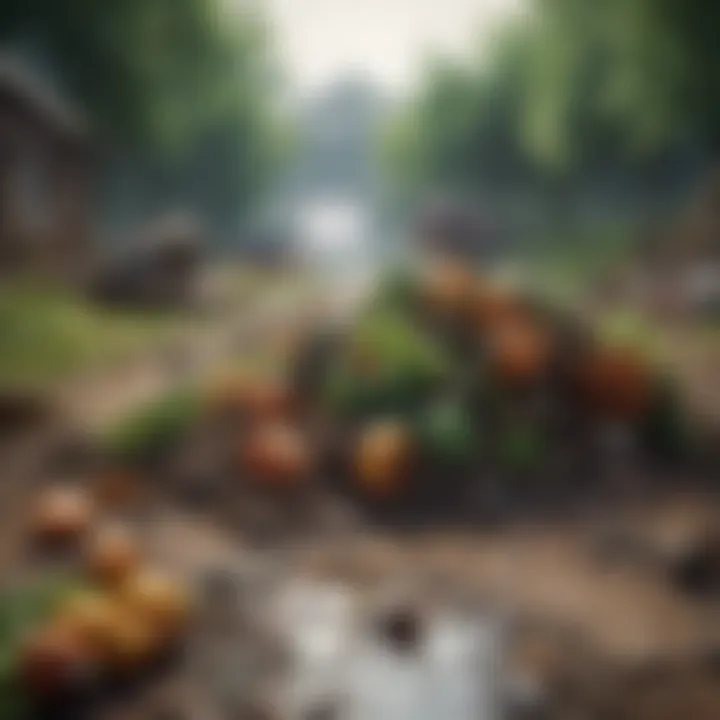
The anaerobic digestion process is a biological method that converts organic materials into biogas in an oxygen-free environment. This process is significant for several reasons.
- Microbial Action: In anaerobic digestion, specific microorganisms break down organic matter. This breakdown releases methane, a valuable energy source.
- Biogas: The biogas produced can be used for heating, electricity production, or as a vehicle fuel. Moreover, the residual material, known as digestate, can be used as a nutrient-rich fertilizer, closing the nutrient loop in agricultural systems.
"Anaerobic digestion plays a crucial role in sustainable waste management, transforming waste into energy and reducing landfill burdens."
- Operational Factors: To maximize efficiency, factors such as temperature, pH, and retention time must be carefully controlled. Optimizing these conditions can significantly enhance the methane yield from organic waste.
Composting vs. Energy Production
Composting and energy production are two distinct pathways for managing organic waste.
- Composting: This is a natural biological process. Organic waste is decomposed in the presence of oxygen, producing humus-like material known as compost. While compost enriches soil, it is not a direct energy source.
- Energy Production through Anaerobic Digestion: In contrast, anaerobic digestion focuses on producing biogas as a renewable energy source. This process captures the energy content of organic waste much more effectively than composting.
Both methods have their place in waste management; however, their end goals differ. Composting supports soil health and carbon sequestration, while anaerobic digestion offers a tangible energy return. This aspect is vital in evaluating which technique to implement, depending on local environmental goals and energy needs.
Technologies for Converting Green Waste to Energy
Green waste energy technologies play an essential role in harnessing organic materials to produce renewable energy. These technologies help to reduce waste disposal issues while simultaneously providing alternative energy sources. The significant advancements in this field underscore the urgency of addressing the growing waste problem with sustainable solutions. Understanding methods of conversion enhances our knowledge of both their environmental benefits and potential challenges.
Thermal Conversion Technologies
Thermal conversion processes transform organic waste into energy through high-temperature applications. These technologies include incineration and gasification. They are crucial because they minimize waste while generating usable energy.
Incineration
Incineration involves burning waste to produce heat. This process contributes significantly to reducing the volume of waste. A key characteristic of incineration is its efficiency in energy recovery. It captures heat generated from the burning process to generate electricity or heat water. This method is popular because it can handle varying types of waste, including non-recyclable materials.
A unique feature of incineration is that it can generate energy while also reducing environmental impacts associated with landfilling. However, it does pose challenges, such as the release of air pollutants and the necessity of stringent emission controls. In the context of this article, incineration is highlighted for its ability to convert waste into energy effectively, despite its complex environmental considerations.
Gasification
Gasification converts organic material into syngas, a mixture of hydrogen and carbon monoxide, through partial combustion. This process is an attractive option for renewable energy because it is capable of producing cleaner energy than incineration. The key characteristic of gasification is its ability to create valuable by-products, including synthetic natural gas and biofuels.
Gasification's unique feature is that it operates at lower temperatures compared to incineration, reducing the formation of harmful emissions. The main advantages include the efficient conversion of biomass and the potential for reducing greenhouse gases. However, gasification systems can be costly to construct and require careful regulation, making them less accessible for some waste management applications.
Biochemical Conversion Technologies
Biochemical conversion technologies utilize biological processes to break down organic materials into energy. The primary methods include fermentation and enzymatic hydrolysis. These processes are vital as they take advantage of natural reactions to create energy and other useful products.
Fermentation
Fermentation is a metabolic process where microorganisms decompose organic matter, producing biogas and bioethanol as by-products. This method contributes significantly to waste management by converting biodegradable materials into renewable energy. The key characteristic of fermentation is its ability to process various organic waste, including food scraps and agricultural residues.
A unique feature of fermentation is its energy output, providing a versatile source of renewable energy that can be used in transportation or heating. This method has advantages, such as lower greenhouse gas emissions and easy integration with existing waste disposal systems. However, challenges exist, including the need for effective pretreatment processes to enhance digestibility.
Enzymatic Hydrolysis
Enzymatic hydrolysis involves using enzymes to catalyze the breakdown of complex organic materials into simpler sugars. This method is gaining importance due to its high conversion efficiency and lesser environmental impact. A key characteristic is its capacity to process lignocellulosic biomass, such as agricultural waste and wood chips.
The unique feature of enzymatic hydrolysis is the specificity of enzymes, allowing for tailored approaches to various biomass types. This results in higher yields compared to traditional methods. Additionally, as it operates at mild conditions, fewer unwanted by-products are produced. However, it can be expensive due to enzyme production costs and the need for large-scale implementation.
Emerging Technologies
Emerging technologies in green waste energy are focusing on innovative approaches to enhance efficiency and reduce costs in waste conversion processes. Advancements in research are likely to lead to new methods of recycling waste into energy, aligning with sustainability goals and enhancing energy self-sufficiency.
Environmental Impacts of Green Waste Energy
Environmental impacts of green waste energy are crucial for understanding how these systems can influence ecological balance and sustainability. This section will delve into key aspects such as carbon footprint reduction, biodiversity, soil health, and air quality. These elements play substantial roles in evaluating both benefits and challenges of green waste energy technologies.
Carbon Footprint Reduction
One of the most significant advantages of utilizing green waste energy is the reduction in carbon footprint. Organic waste, when managed improperly, contributes to greenhouse gas emissions, particularly methane. Methane is a potent gas, approximately 25 times more effective at trapping heat in the atmosphere than carbon dioxide over a 100-year period.
By converting green waste through processes like anaerobic digestion or incineration, these emissions can be minimized significantly. Waste-to-energy facilities not only prevent landfill methane emissions but also capture released carbon, transforming it into usable energy like electricity or heat. According to studies, implementing waste-to-energy strategies can reduce overall greenhouse gas emissions by a substantial percentage.
Biodiversity and Soil Health
Green waste energy can also positively impact biodiversity and soil health. When organic materials are composted or properly processed, they enrich the soil, leading to improved nutrient cycling and reduced need for chemical fertilizers. Healthy soil contributes to diverse ecosystems, supporting various plant and animal species.
The regenerative capacity of composting returns vital organic matter to the earth, which fosters not only plant growth but also enhances the resilience of microbial communities. This is essential for maintaining biodiversity. Additionally, the use of biogas for energy supports sustainable farming practices by providing local farmers with an alternative to fossil fuels, further promoting biodiversity.
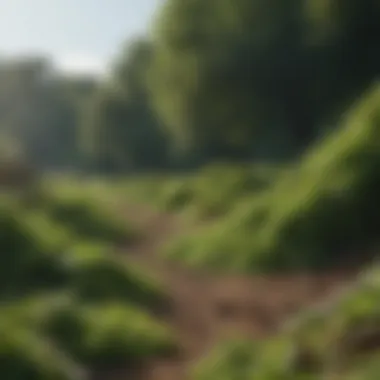
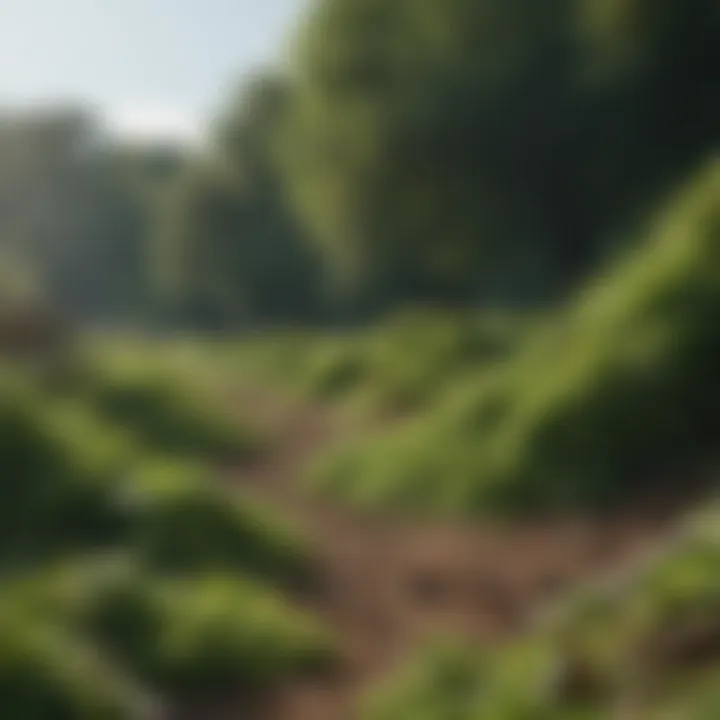
Air Quality Concerns
While there are clear advantages, it's important to also consider air quality concerns. Some waste-to-energy technologies may release pollutants if not managed correctly. Incineration, for instance, can emit harmful substances, including dioxins and particulate matter. These pollutants can degrade air quality and pose health risks to surrounding communities.
To mitigate these concerns, strict regulations and advanced filtration technologies need to be employed. Continuous monitoring of emissions is essential to ensure compliance with environmental standards. When such measures are integrated, the impacts of green waste energy on air quality can be managed effectively, aligning with the overall goals of environmental protection.
"Effective waste management through green waste energy promotes healthier ecosystems while addressing climate change challenges."
Finale
In summary, the environmental impacts of green waste energy are multifaceted. They encompass both beneficial effects, like reducing carbon footprints and improving soil health, as well as challenges such as air quality concerns. Addressing these considerations is vital for the successful implementation and future development of green waste energy technologies.
Economic Aspects of Green Waste Energy
The economic aspects of green waste energy represent a crucial factor in its adoption and success. As the world increasingly seeks sustainable solutions to waste management and energy generation, understanding the financial implications is essential. This section examines several key elements regarding the economic viability of green waste energy solutions, including costs, benefits, government supports, and current market trends.
Cost-Benefit Analysis of Waste-to-Energy Systems
Conducting a cost-benefit analysis is vital in determining the practicality of waste-to-energy systems. Such systems have various costs, including initial capital required for technology, operational expenses, and maintenance costs. However, they offer significant benefits, which make them an appealing consideration.
Key Costs:
- Capital Investment: Setting up facilities for green waste energy requires substantial investment. Technology varies greatly in price, from anaerobic digesters to thermal conversion plants.
- Operational Costs: Ongoing costs include labor, logistics, and energy consumption in the processing of waste.
- Regulatory Compliance: Adhering to environmental and operational regulations may incur additional costs.
Key Benefits:
- Revenue Generation: Selling energy produced from waste can be profitable, especially with the rise of renewable energy markets.
- Waste Reduction: Maximizing waste management efficiency leads to lower disposal costs.
- Environmental Benefits: Reduced greenhouse gas emissions may lead to savings through carbon credits or compliance rebates.
Overall, a detailed cost-benefit analysis helps stakeholders determine whether pursuing green waste energy conversion is a financially sound investement.
Government Policies and Incentives
Government policies and financial incentives play a decisive role in promoting green waste energy systems. Various forms of support can mitigate the financial burdens of implementation. This includes grants, tax credits, and subsidies aimed at boosting development in renewable energy sectors.
Support Mechanisms:
- Grants and Loans: Many governments provide financial assistance to start green waste projects. This can greatly reduce entry barriers.
- Tax Incentives: Tax credits and deductions for renewable energy production can encourage companies to invest in green technologies.
- Regulatory Support: Policies mandating waste-to-energy adoption in waste management practices influence industry trends.
Successful implementation of these policies fosters an environment conducive to investment in green waste energy technologies, leading to sector growth.
Market Trends in Renewable Energy
Understanding current market trends is essential to grasp the broader economic landscape surrounding green waste energy. Global awareness about climate change and increased demand for renewable energy sources are shaping market dynamics.
Current Trends:
- Rising Demand for Renewable Energy: Many countries have set ambitious targets for carbon neutrality, prompting investments in renewable energy, including waste-to-energy.
- Technological Advancements: Improvements in conversion technologies make green waste energy solutions more efficient and less costly.
- Investment Growth: There is a notable increase in venture capital investing in clean energy startups and established companies focusing on waste management and energy production.
- Circular Economy Adoption: More businesses are integrating circular economy practices, leading to an increased supply of green materials suitable for energy production.
In summary, the economic aspects of green waste energy involve assessing costs and benefits, leveraging government support, and analyzing market conditions. These factors collectively inform stakeholders about the viability and potential of green waste energy systems in today's economy.
Barriers to Implementation
The journey towards effective green waste energy solutions is littered with a variety of challenges that hinder the process. Understanding these barriers is key to moving forward in promoting sustainability and renewable energy. By acknowledging the obstacles, stakeholders can devise strategies to overcome them, making green waste energy a more feasible option in the global push for cleaner energy.
Technological Challenges
One of the primary barriers to implementing green waste energy systems is the technological challenges involved. Many existing technologies for converting waste to energy are still developing and may not be advanced enough for widespread use. For instance, certain methods like gasification require high temperatures and specific conditions to operate efficiently. Additionally, lack of standardization among technologies can lead to complications in scalability and integration into existing waste management systems.
The need for significant investment in research and development also looms large. Many companies and governments can hesitate to invest due to the perceived risks associated with funding unproven technologies. This needs careful consideration, as technological advancements are crucial for improving efficiency and lowering costs in green waste energy systems.
Public Perception and Acceptance
Another notable barrier is public perception and acceptance of waste-to-energy initiatives. Many individuals associate waste incineration projects with negative environmental impacts. This resistance can stem from misconceptions about air quality and pollution, leading to public opposition. When communities express concerns, it can create significant setbacks for projects that rely on local support for approval and funding.
Educating the public about the benefits of green waste energy is essential. Clearly communicating how modern technologies minimize emissions and contribute to recycling efforts can help alleviate fears. Furthermore, involvement in community discussions might foster a sense of ownership and help promote acceptance.
Regulatory Hurdles
Lastly, regulatory hurdles can impede the progress of green waste energy projects. The regulatory landscape governing waste management and energy generation can be intricate and restrictive. Compliance with stringent environmental regulations often requires extensive documentation, which can delay project implementation.
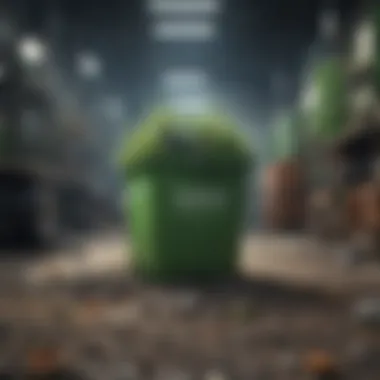
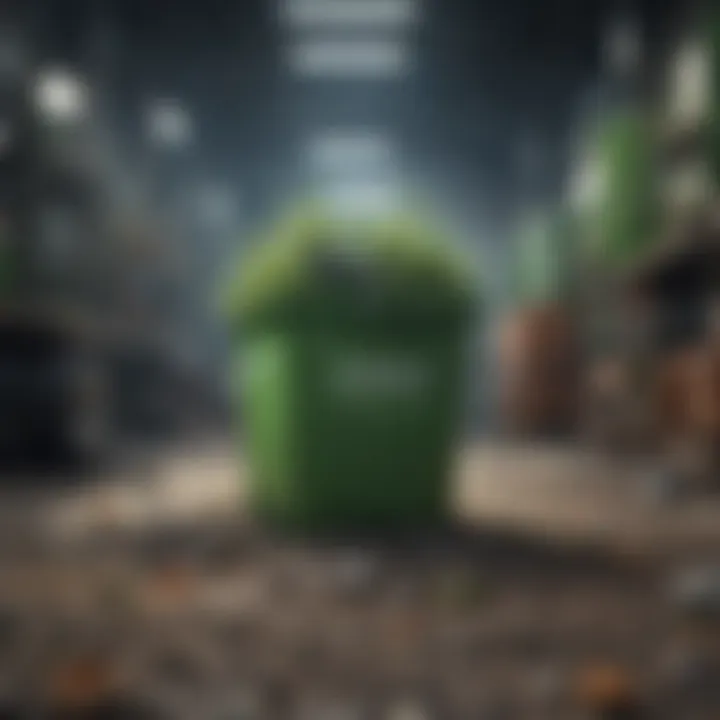
Moreover, varying regulations across regions create challenges for companies aiming to operate in multiple jurisdictions. This inconsistency can lead to increased costs and complexity, discouraging investment in green waste initiatives. Advocating for streamlined regulations could help minimize these barriers and encourage a more conducive environment for green waste energy.
"Understanding the barriers to implementation is crucial in identifying pathways for sustainable energy solutions. Without addressing these issues, green waste energy remains underutilized."
In summary, tackling the barriers to implementation involves a concerted effort from technological advancements, public education, and regulatory reform. Only through addressing these areas can we fully realize the potential of converting green waste into valuable energy.
Future of Green Waste Energy
The future of green waste energy is significant not only for enhancing waste management but also for addressing broader sustainability concerns. As societies strive for cleaner energy sources, green waste energy emerges as a critical component. This section will explore various aspects that shape its future, including innovative research, integration with circular economy principles, and the potential for global adoption.
Innovative Research and Development
Innovative research is pivotal to advancing green waste energy technologies. New methods and improvements are being developed continuously. Research focuses on optimizing existing processes like anaerobic digestion and enhancing energy extraction from waste. This involves studying diverse waste types to determine their most efficient conversion methods.
Additionally, researchers are investigating advanced materials and techniques to improve energy yield. For example, researchers are exploring thermochemical conversion processes to convert biomass into syngas more effectively. By developing more cost-effective, efficient technologies, future breakthroughs can enhance green waste energy's viability.
Integration with Circular Economy Models
The concept of a circular economy aligns closely with the principles of green waste energy. In circular models, waste is minimized, and resources are reused. Green waste energy supports this by converting organic waste into usable energy, thus closing the loop in waste management. This approach not only reduces landfill waste but also contributes to a more sustainable resource chain.
Governments and organizations are increasingly recognizing the potential benefits of integrating green waste energy solutions within circular economy frameworks. This integration can lead to improved resource efficiency and reduced environmental footprint. It fosters a culture of sustainability wherein businesses, communities, and policymakers collaborate to achieve shared objectives.
Potential for Global Adoption
The potential for global adoption of green waste energy technologies is vast. Many countries face the pressing challenge of waste management and energy demands. By adopting green waste energy, nations can address both effectively. Countries with robust agricultural sectors can especially benefit by transforming agricultural residues into energy.
Moreover, international cooperation can accelerate knowledge sharing and technology transfer. Developing nations may require financial and technical assistance to implement these solutions. As global awareness of environmental issues continues to grow, drives for sustainable energy solutions will likely intensify. This trajectory suggests a promising future for green waste energy on a global scale.
"Green waste energy is not just a waste management solution; it's a pathway to sustainable development across the globe."
Case Studies
The examination of case studies in the realm of green waste energy serves as a fundamental component of this discourse. Case studies offer direct, real-world examples of how various methodologies and technologies are applied in the field. They provide insights into successful practices alongside lessons learned from challenges faced. By analyzing these instances, stakeholders can gain a clearer understanding of the viability and impact of implementing green waste energy systems.
Successful case studies illuminate effective strategies and highlight the benefits of harnessing green waste for energy production. They encapsulate innovations, community engagement, and the integration of technologies into existing infrastructures. These shared experiences can guide future projects, demonstrating scalability and adaptability to different contexts. Moreover, the challenges confronted by these case studies offer critical reflections for future implementations, allowing stakeholders to preemptively navigate potential hurdles.
"Case studies not only showcase successful projects but also provide a roadmap for overcoming obstacles in green waste energy adoption."
Successful Implementation in Urban Areas
Urban environments present unique opportunities and challenges for green waste energy initiatives. High population density generates significant quantities of organic waste, creating a substantial feedstock for energy conversion processes. Cities like San Francisco have successfully implemented programs that prioritize composting and anaerobic digestion, effectively transforming waste into energy and compost.
Key factors contributing to successful implementation in urban settings include:
- Comprehensive Waste Management Systems: Effective integration of waste collection, sorting, and treatment ensures maximum recovery of organic material.
- Public Engagement: Educational campaigns and community involvement are vital in fostering public acceptance and participation.
- Technological Advancements: The utilization of smart technologies aids in optimizing waste collection and processing systems, enhancing efficiency.
The case of San Francisco stands as a benchmark. The city's commitment to diverting waste from landfills led to establishing a robust composting program. As a result, a significant proportion of residential and commercial waste is now converted into energy and compost, reducing landfill use and greenhouse gas emissions. Such models provide a practical guide for other urban areas contemplating similar initiatives.
Rural Waste Management Approaches
Rural areas face different waste management dynamics compared to urban counterparts. The sparse population often translates to lower waste volumes per capita, yet the agricultural nature of these regions provides ample organic waste resources. Successful rural waste management approaches leverage local characteristics to foster sustainable energy production.
- Localized Solutions: Rural communities benefit from tailored anaerobic digesters that process manure and crop residues, converting them into biogas and organic fertilizers.
- Collaboration: Partnerships between farms, local governments, and energy companies can enhance resource sharing and economic viability.
- Education and Training: Providing training on sustainable practices ensures community members are equipped with the knowledge necessary to participate actively in management efforts.
One notable example can be seen in Denmark, where agricultural operations incorporate anaerobic digestion systems into their practices. These systems not only generate biogas for energy but also provide high-quality fertilizers for crops, thus closing the nutrient loop. Such instances illustrate the potential for rural areas to capitalize on their natural waste streams, leading to both energy production and improved agricultural sustainability.
By drawing from these case studies, stakeholders can acknowledge the adaptability and scalability of green waste energy technologies across diverse settings. This knowledge can inspire future initiatives, optimize resource utilization, and ultimately lead to more sustainable waste management practices.
The End
The importance of examining green waste energy becomes increasingly clear as we face escalating environmental challenges. This conclusion synthesizes insights gleaned throughout the article, reinforcing the necessity for a shift in how we perceive and manage organic waste. Green waste energy initiatives offer multifaceted benefits, not only addressing waste management issues but also contributing to renewable energy sources.
Key Insights
Green waste energy stands at the intersection of sustainability and innovation. Here are some critical takeaways:
- Sustainable Waste Management: Green waste energy provides viable alternatives to traditional waste disposal methods. By harnessing organic materials, we can significantly reduce landfill contributions and mitigate environmental pollution.
- Renewable Energy Contribution: Technologies such as anaerobic digestion and gasification transform waste into energy, providing renewable power that can stabilize energy grids.
- Economic Viability: Investment in green waste energy processes presents multiple economic opportunities. The cultivation of local energy solutions can strengthen community resilience and create jobs.
- Environmental Protection: Utilizing green waste energy helps reduce greenhouse gas emissions, supporting climate change mitigation efforts. The positive impact on biodiversity and soil health adds another layer of environmental preservation.
Call for Action
To realize the full potential of green waste energy, proactive steps are needed across various sectors:
- Policy Advocacy: Stakeholders, including educators, researchers, and community leaders, must advocate for supportive policies and incentives that promote green waste energy solutions.
- Public Awareness Campaigns: Raising awareness about the benefits and applications of green waste energy can shift public perception. Education can spur community engagement in local waste management initiatives.
- Research Funding: Increased funding for research into innovative technologies and processes is crucial. Our understanding of green waste energy can significantly advance with dedicated investment in this area.
- Partnership Development: Collaboration among governments, businesses, and non-profits can foster the growth of green waste energy projects, capitalizing on shared resources and knowledge.
"The transition to a sustainable future relies on how effectively we can change our approach to waste management and energy production."
By embracing green waste energy solutions, we not only move closer to achieving a circular economy but also invest in a sustainable and resilient future.