High Output Wind Turbines: Innovations and Future Trends
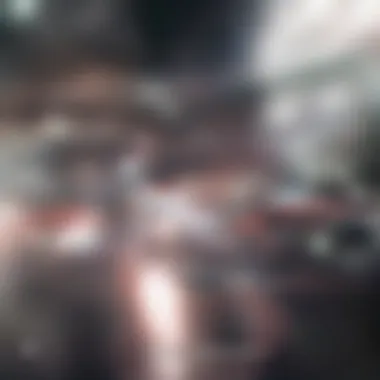
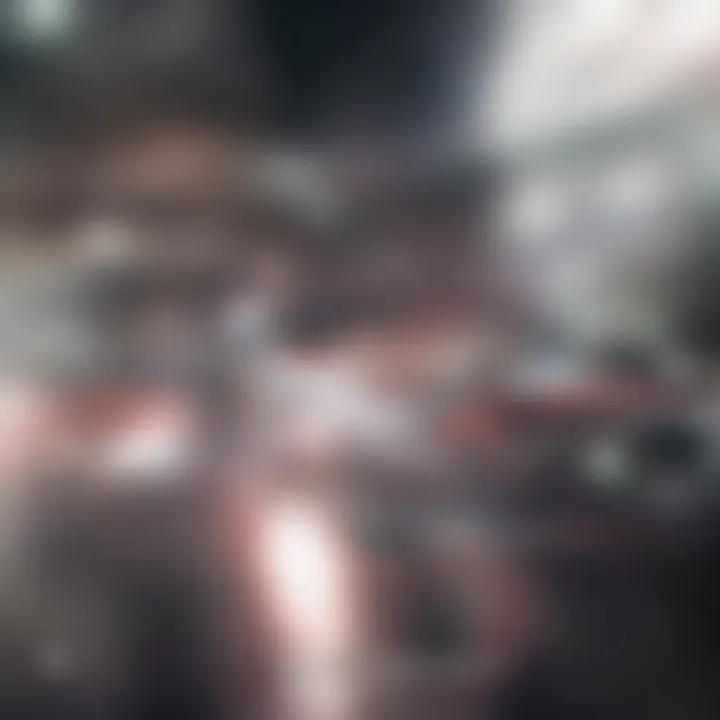
Intro
High output wind turbines represent a significant evolution in the landscape of renewable energy technology. The global demand for clean energy challenges engineers and researchers to optimize turbine designs, boost output efficiency, and integrate these systems into existing energy infrastructures. Understanding the advancements in turbine technology and their applications reveals their immense potential.
Research Context
Background and Rationale
As climate change concerns rise, the need for sustainable energy solutions becomes even more pressing. High output wind turbines play a pivotal role in this scenario, effectively harnessing wind energy to produce significant amounts of electricity. The rationale behind focusing on this sector is mainly to explore how advancements in design and technology can improve energy yield and reduce reliance on fossil fuels.
Literature Review
Numerous studies have detailed the innovation in turbine design, including larger rotor sizes, vertical axis configurations, and advanced materials that enhance durability and performance. A considerable body of research has analyzed the integration of wind energy systems into electrical grids. These studies highlight the importance of energy storage solutions, such as batteries, to stabilize output amid fluctuating wind patterns. Insights from various academic works and industry reports will be examined to provide a rounded perspective on the advancements and current applications.
"The integration of high output wind turbines is essential for achieving a sustainable energy future."
Methodology
Research Design
This article employs a qualitative research design leveraging existing literature, industry reports, and case studies of high output wind turbine installations. This approach allows for an in-depth analysis of the trends, challenges, and innovations within the sector.
Data Collection Methods
Data was primarily gathered through academic journals, market analysis reports, and interviews with industry experts. These sources provide a comprehensive view of the technological advancements and practical applications of high output wind turbines.'
Prelude to High Output Wind Turbines
The topic of high output wind turbines is increasingly significant in today's context of renewable energy. As demand for sustainable and efficient energy sources grows, understanding and advancing high output wind turbines become essential. These systems can significantly augment energy production, thus playing a vital role in moving toward cleaner energy practices. The advancements in turbine technology enhance not only energy output but also efficiency and reliability, which is crucial for meeting energy requirements across various sectors.
Transporting us into the spectrum of renewable technologies, this article seeks to explore several crucial aspects of high output wind turbines. The following sections focus on their definition, overview, historical milestones, technological advancements, and more. An analysis of applications reveals their versatility, while integration within power systems offers insights into how they align with modern energy needs. Important challenges such as economic barriers and regulatory constraints are also discussed to provide a well-rounded perspective.
Moreover, as global markets shift and adopt renewable solutions, examining future directions presents the opportunity to identify trends that could define the next era of wind energy.
Definition and Overview
High output wind turbines refer to systems designed to maximize electricity production from wind. These turbines typically include advanced technology that enhances their operational capacity, making them suitable for large-scale energy generation. Characterized by larger rotor diameters and taller towers, high output turbines harness greater wind energy compared to traditional models. They represent a crucial innovation that meets the demand for higher efficiency and reliability in energy production.
In essence, high output wind turbines are at the forefront of wind energy evolution. They are not only designed to facilitate the generation of electricity but also to optimize energy use from wind resources effectively. A clear understanding of what constitutes high output turbines is essential for further discussions in this article.
Historical Development
The journey of wind turbines has transformed considerably over the decades. Initially, windmills served basic needs like grinding grain and pumping water. This focus on mechanical energy shifted in the late 19th century, with pioneers creating the first electric wind turbines.
The historical landscape of wind energy saw substantial changes by the late 20th century. Technological advancements allowed for the construction of larger turbines capable of producing more electricity. The introduction of computerized control systems enhanced operational efficiency and reliability.
In the early 2000s, a new wave of development occurred, leading to the design of high output turbines, specifically built to meet energy needs in various environments, including offshore locations. These advancements were driven by breakthroughs in materials science and engineering, contributing to the modern designs we see today.
The evolution of high output wind turbines mirrors the growing global focus on sustainable energy solutions. As societies look toward renewable resources to combat climate change, understanding this trajectory becomes paramount, ensuring we are prepared to embrace innovative strategies for energy production.
Technological Advancements
Technological advancements play a critical role in the evolution and efficiency of high output wind turbines. These innovations enhance energy production, improve reliability, and reduce the cost of energy generation. As the demand for renewable energy grows, it becomes essential to harness every possible improvement in turbine design and functionality. The focus on such advancements has led to better performance, increased capacity, and overall sustainability of wind energy systems.
Design Innovations
Horizontal versus Vertical Axis Turbines
Horizontal axis turbines (HATs) remain the most popular type of wind turbine in commercial applications. They are generally more efficient at capturing wind energy due to their design, allowing them to rotate more efficiently with the wind’s direction. The key characteristic of HATs is their elongated structure with blades positioned horizontally, which maximizes the area available for capturing wind energy.
In contrast, vertical axis turbines (VATs) offer unique benefits in certain contexts. These turbines can capture wind from any direction without requiring a yawing mechanism. Their unique feature is the vertical arrangement, which allows them to be placed in challenging environments with turbulent winds. However, they are generally less efficient than horizontal axis types in most conditions. HATs are often deemed better for utility-scale projects, while VATs might suit urban settings better.
Blade Design and Materials
Blade design and materials directly impact the efficiency and performance of wind turbines. Advances in aerodynamics have led to more streamlined blade shapes that can capture wind more effectively. One key characteristic is the usage of composite materials, which are lighter and stronger than traditional options. This reduces the weight of the blades, allowing for longer designs that can reach higher wind speeds.
The unique feature of advanced blade materials lies in their resilience against environmental factors, such as corrosion and fatigue. However, there are disadvantages related to costs and recycling those materials at the end of the blade's lifecycle. The innovative designs, combined with improved materials, significantly contribute to maximizing energy production in high output wind systems.
Control Systems and Software
Control systems and software are vital components for managing the operational effectiveness of wind turbines. These systems monitor and adjust turbine operations to optimize energy capture. The innovative control algorithms can enhance performance by adapting to changing wind conditions, which ensures the turbine operates within its optimal capacity.
An important feature of these systems is their integration with smart grid technologies. This synergestic relationship further improves stability and efficiency in the energy supply chain. Nevertheless, the reliance on complex technology also brings vulnerabilities, such as potential system failures or cybersecurity risks.
Material Science Impact
Lightweight Materials
The use of lightweight materials has transformed the construction of wind turbines. Generating less weight means turbines can be built taller and with larger rotor diameters. This directly contributes to increased energy output, as taller turbines can access higher wind speeds. The key characteristic of lightweight materials is that they reduce the load on the turbine structure and support systems.
Thus, maintenance can also be simplified due to less wear over time. Additionally, lighter components can lead to lower transportation costs and simplified logistics during installation. However, while they offer advantages in performance, the long-term durability of some lightweight materials can be a concern in harsh environmental conditions.
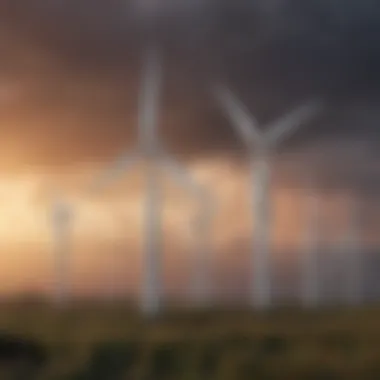
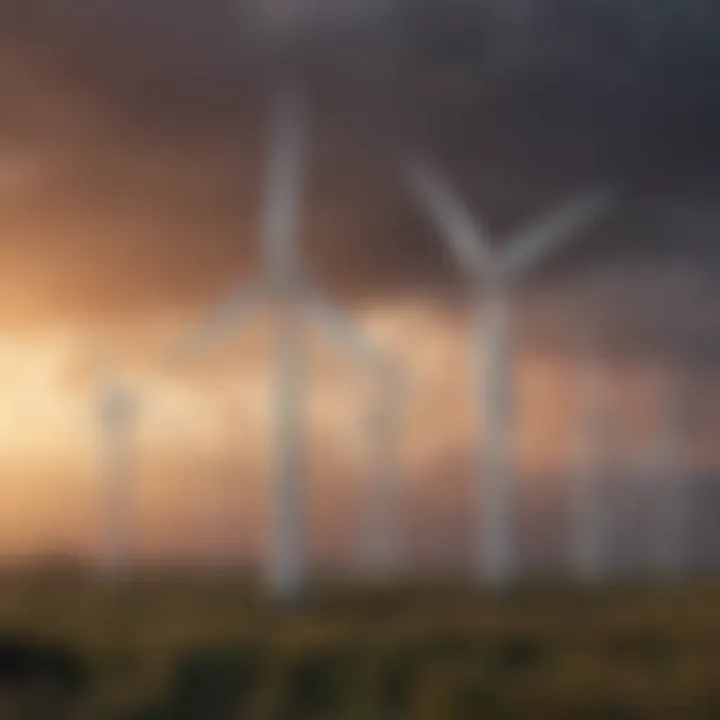
Durability and Performance
Durability measures the robustness of wind turbine components against external pressures such as extreme weather. Innovations in materials science have led to components designed for higher durability, which is crucial in maximizing the lifespan and effectiveness of the turbines. A significant characteristic of enhanced durability is the development of materials that resist wear and tear, especially in critical components like bearings and gears.
The unique advantage is a lower frequency of maintenance and replacements, which can significantly reduce operational costs. However, higher initial costs associated with developing and implementing durable materials remain a consideration.
Sustainability in Production
Sustainability in production is a vital issue in the wind energy sector. Advances in manufacturing processes now allow for less environmental impact during the production of turbine components. The key characteristic lies in the adoption of cleaner methods and materials that reduce emissions.
This increase in sustainable practices addresses public concerns about the ecological footprint of renewable energy technologies. However, sustainable practices may sometimes involve higher costs, which can impact the overall economics of wind projects. Balancing cost with environmental responsibility is essential as the industry seeks to expand.
"Technological advancements in high output wind turbines are crucial to meeting global energy demands sustainably."
Efficiency Metrics
Efficiency metrics are critical in understanding the performance and effectiveness of high output wind turbines. These metrics provide insight into how well turbines convert wind energy into usable electricity. Assessing these efficiency parameters is essential for optimizing turbine designs, operational strategies, and integrating them into power systems. By focusing on the specific aspects of power output calculations and capacity factors, stakeholders can make informed decisions that impact the overall success of wind energy projects.
Power Output Calculations
Power output calculations serve as a foundation for evaluating the energy production potential of high output wind turbines. These calculations consider several variables, including wind speed, rotor diameter, and turbine efficiency. By applying power equations, such as the Betz limit, engineers can estimate the maximum energy that can be captured from the wind. Accurate calculations are vital as they determine the anticipated performance in different wind conditions. The efficiency of a turbine is heavily reliant on how well it can harness wind energy, making precise calculations essential for project feasibility assessments.
Capacity Factors
Capacity factors offer a deeper understanding of how effectively turbines operate compared to their maximum potential output. A high capacity factor indicates that a turbine is producing close to its rated power output over time, which is beneficial for economic viability and energy reliability.
Seasonal Variability
Seasonal variability is a significant factor impacting capacity factors for wind turbines. In many regions, wind patterns change with the seasons, affecting energy production. For instance, a location might experience stronger winds during winter months, leading to higher power generation. This characteristic makes seasonal variability a crucial consideration in turbine site selection and energy forecasting. Planning for these fluctuations can enhance the resilience and efficiency of energy production throughout the year.
Geographic Considerations
Geographic considerations significantly influence the performance of wind turbines. Factors such as terrain, altitude, and proximity to coastlines play vital roles in wind patterns. Locations with consistent wind flow will usually result in better capacity factors. Assessing these geographic features is important because it informs decisions about where to install turbines. Additionally, some regions experience competing land uses, which can limit suitable sites for installation. Therefore, understanding geography is essential for optimizing turbine deployment and maximizing energy capture.
Comparative Analysis with Traditional Turbines
A comparative analysis with traditional turbines reveals key insights into high output turbine performance. Traditional turbines often have lower efficiency and capacity factors compared to their modern counterparts. High output turbines are designed with advanced technologies, enabling them to extract more energy from the wind under similar conditions. This analysis highlights substantial improvements in blade design, materials, and control systems that contribute to enhanced energy harvesting capabilities. Understanding the differences not only helps in promoting high output wind turbines but also aids in advancing standards and practices within the wind power industry.
Applications of High Output Wind Turbines
The integration of high output wind turbines into various energy sectors is pivotal for advancing renewable energy efforts. The importance of this topic lies in its capacity to illustrate how these turbines can serve diverse applications, ranging from agricultural enhancements to large-scale power generation. Each type of installation has distinct benefits, contributing to overall energy efficiency, sustainability, and economic growth.
Onshore Installations
Farm Applications
Farm applications of high output wind turbines are increasingly popular in the agricultural sector. They provide farmers with a unique opportunity to generate their own energy, which can significantly reduce costs over time. A key characteristic is the adaptability of these turbines to various terrains and farm sizes. This flexibility makes them a beneficial choice for renewable energy strategies.
A notable feature of farm applications is the relatively low initial investment required compared to larger scales of deployment. Farmers can start with smaller turbines and scale up as needed. However, one disadvantage might be the dependency on wind consistency, which can vary by region. Nevertheless, the costs saved can offset these concerns, demonstrating a solid return on investment.
Community Energy Projects
Community energy projects represent a collaborative approach to harnessing wind energy. These initiatives empower local communities to take control of their energy sources. A key characteristic of community projects is the potential for shared financial and environmental benefits. They often foster a sense of solidarity and shared responsibility among community members.
Communities can uniquely tailor these projects based on local needs and resources. The main advantage is the reduction of energy poverty, as locals gain access to sustainable and affordable energy. However, challenges might include navigating local regulations and securing initial funding. These barriers can vary significantly based on location but can often be overcome through collaboration.
Utility Scale Deployments
Utility scale deployments are essential in meeting high energy demands, particularly in urban areas. These large setups are characterized by their capacity to produce extensive amounts of electricity. They play a vital role in integrating renewable sources into the broader energy grid.
The unique feature of utility-scale deployments is their ability to provide reliable and large quantities of renewable energy can effectively replace fossil fuels. This can significantly decrease carbon emissions. One of the disadvantages includes higher initial capital investment and construction time compared to smaller installations. Nonetheless, their potential for sustainable energy production makes them an attractive solution for future energy needs.
Offshore Installations
Site Selection Criteria
The criteria for selecting sites for offshore wind turbine installations are critical for maximizing efficiency and minimizing costs. Key considerations include wind patterns, water depth, and distance from shore. This careful selection is vital to ensure that turbines operate at optimal capacity.
A prominent characteristic of site selection is the potential to harness stronger winds available offshore. This can result in higher energy production compared to onshore installations. However, logistical challenges such as transportation and installation can arise due to the remote locations. Adequate planning can mitigate these disadvantages, allowing for successful deployments.
Construction and Maintenance Challenges
Construction and maintenance challenges represent significant aspects of offshore turbine projects. These projects often involve intricate logistical planning and sophisticated engineering solutions. A key characteristic of these challenges is the potential for high costs and complex operational requirements.
The unique features of offshore operations include specialized vessels and equipment to ensure safety and efficiency. Although the costs can be considerable upfront, the long-term benefits include higher energy output and reduced land usage onshore. Understanding these challenges is imperative for successful project completion.
Environmental Impact Assessments
Environmental impact assessments (EIAs) are vital for understanding and mitigating the impacts of offshore wind projects. They evaluate the potential ecological consequences of installing high output turbines in marine environments. A key characteristic of EIAs is their ability to inform regulatory agencies and stakeholders of potential risks before projects commence.
The unique feature of comprehensive EIAs includes their focus on preserving marine life. This is crucial, given the sensitivity of coastal ecosystems. While conducting thorough assessments can prolong project timelines, the benefits often outweigh these delays. Properly conducted EIAs enhance sustainability and public acceptance of offshore wind energy initiatives.
Integration within Power Systems
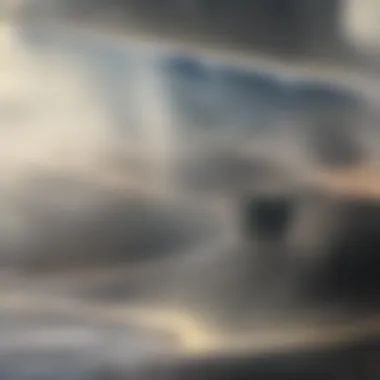
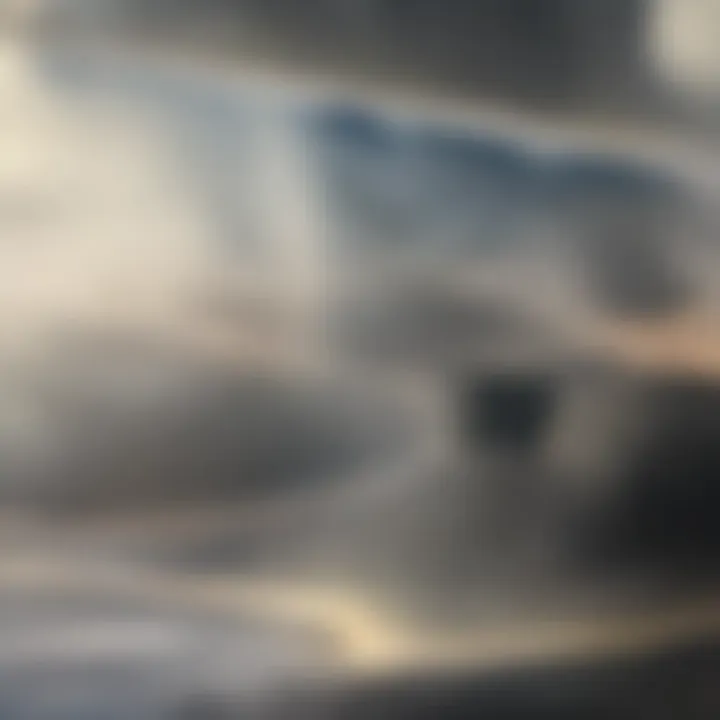
Integration within power systems is crucial for maximizing the potential of high output wind turbines. As renewable energy sources continue to grow, the need for seamless connectivity becomes more apparent. High output wind turbines must effectively integrate into existing energy networks for optimal efficiency and reliability. Proper integration not only enhances overall system performance but also contributes to a resilient energy infrastructure.
Connecting wind energy effectively to the grid involves several considerations. These include compatibility with smart grid technologies, energy storage solutions, and demand response strategies. Each aspect plays a significant role in ensuring that renewable sources can complement traditional energy systems, ultimately leading to a greener and more sustainable energy future.
Grid Connectivity
Smart Grid Technologies
Smart grid technologies represent a significant advancement in energy management. They enhance efficiency and enable better data exchange between consumers and energy providers. One of the key characteristics of smart grids is their ability to optimize energy distribution in real time. This capability allows high output wind turbines to function in harmony with the energy demands of the grid, resulting in minimized waste and maximized output.
The unique feature of smart grid technologies lies in their integration of communication tools with energy distribution systems. This leads to improved monitoring and management capabilities. However, the implementation of smart grids often requires substantial investment and thorough planning. Balancing the costs with potential long-term benefits is crucial for stakeholders.
Energy Storage Solutions
Energy storage solutions play a vital role in stabilizing energy supply from high output wind turbines. They allow for the capture and storage of excess energy generated during peak production times. One key characteristic of energy storage systems is their ability to supply energy during periods of low wind. This ensures that energy supply remains consistent, addressing one of the most significant challenges of wind energy: intermittency.
A unique feature of contemporary energy storage solutions is the advancement in battery technologies, particularly lithium-ion batteries. These systems offer faster response times and higher energy density. However, the initial costs associated with energy storage deployment can be considerable, and managing the lifecycle of storage systems remains a challenge.
Demand Response Strategies
Demand response strategies are effective ways to adjust energy consumption based on supply conditions. They create a balance between energy demand and the generation capacity of high output wind turbines. A notable characteristic of demand response is its flexibility, allowing consumers to reduce or shift their energy usage during peak demands.
These strategies typically involve incentivizing users to alter their energy consumption habits. Demand response stands out because it can help integrate renewable energy by reducing reliance on fossil fuel-based sources during critical times. However, educating consumers and ensuring participation can present challenges in this approach.
Hybrid Energy Systems
Wind and Solar Integration
Integrating wind and solar energy sources can create hybrid energy systems that significantly enhance energy reliability. Wind and solar integration combines the strengths of both technologies. The variability of wind and solar generation can counterbalance each other, leading to more stable energy outputs. A key characteristic of this integration is the complementary nature of wind and solar resources across different times of the day and seasons.
The uniqueness of wind and solar integration lies in its potential to optimize land use and reduce the overall carbon footprint. However, managing the interdependencies between systems can pose logistical challenges, as well as require careful planning.
Combined Heat and Power Solutions
Combined heat and power, or CHP systems, utilizes the heat produced during electricity generation to provide heating for buildings or processes. This creates a more efficient overall energy output. A key characteristic of CHP is its ability to capture and reuse thermal energy that would otherwise be wasted in traditional systems.
The unique aspect of CHP systems is their capability to enhance energy efficiency by converting fuel into both electricity and usable heat. Despite their advantages, the initial investment and technical complexity can deter deployment in certain settings, particularly in small-scale applications.
Geothermal and Wind Synergies
Geothermal and wind synergies present an interesting opportunity for renewable energy systems. By combining geothermal energy with wind power, it is possible to create a more consistent energy output. A primary characteristic of this synergy is the ability of geothermal systems to provide a stable baseline energy supply while wind energy contributes during optimal conditions.
The unique feature here is the reliability of geothermal energy as a resource that operates independently of weather conditions. Nevertheless, the geographic limitations of geothermal resources may limit widespread application in certain regions.
Challenges in Deployment
The deployment of high output wind turbines presents several challenges that are crucial for the widespread implementation of this renewable energy technology. Understanding the barriers provides a clear view of the landscape in which these turbines operate. These challenges primarily stem from economic barriers and regulatory constraints. Both areas must be navigated to maximize the potential of high output wind energy.
Economic Barriers
Economic barriers significantly affect the large-scale adoption of high output wind turbines. Each component of these economic challenges plays a role in the viability and attractiveness of investing in wind energy.
Initial Investment Costs
Initial investment costs represent one of the largest hurdles for developers and investors considering high output wind turbine projects. These costs include expenses related to the purchase of turbines, installation, and connection to grids. Importantly, securing capital for these projects can be challenging.
- The key characteristic of initial investment costs is their substantial magnitude. High upfront costs can deter potential investors, creating a major obstacle for project initiation.
- The unique feature of these costs is that they are often offset by long-term savings on operational expenses and energy generation. However, the high initial requirement remains a concern in investment decisions.
Return on Investment Analysis
Return on investment (ROI) analysis is vital for understanding the financial benefits of installing high output wind turbines. Assessing ROI provides insight into profitability over time, which is crucial for stakeholders.
- The key characteristic of ROI analysis is its capability to forecast potential revenue generated from the turbine operation against installation costs.
- A unique feature of ROI analysis is its ability to vary widely depending on local conditions, including wind availability and market prices. This variability can lead to skepticism among potential investors.
Funding and Grants
Funding and grants are essential mechanisms that can alleviate the financial barriers associated with deploying high output wind turbines. Various public and private organizations offer financing options designed to support renewable energy initiatives.
- The key characteristic of funding and grants is their ability to reduce the financial burden on developers, making projects more feasible.
- However, the unique feature of access to these financial resources can often depend on regulatory incentives and political will. This unpredictability can result in project delays and increased uncertainty for developers.
Regulatory Constraints
Regulatory constraints significantly affect how efficiently high output wind turbines can be deployed. They often dictate the terms under which projects can operate, impacting timelines and costs.
Permitting Processes
Permitting processes are critical path elements that impact the deployment of wind turbines. They determine the legal and public review stages that projects must undergo before they can start.
- The key characteristic of permitting processes is their complexity, as it often involves multiple stakeholders, including local, state, and federal organizations.
- A unique feature of these processes is that they can lead to long delays, frustrating project developers and hindering timely project completion.
Zoning Laws
Zoning laws govern the spatial arrangement and land use around proposed wind turbine installations. These regulations can heavily influence project viability and location decisions.
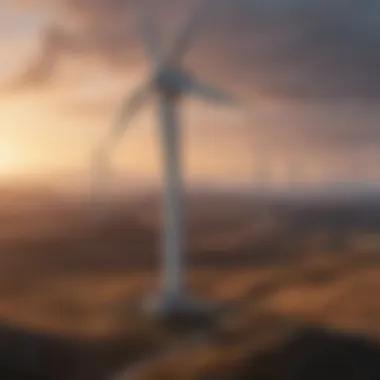
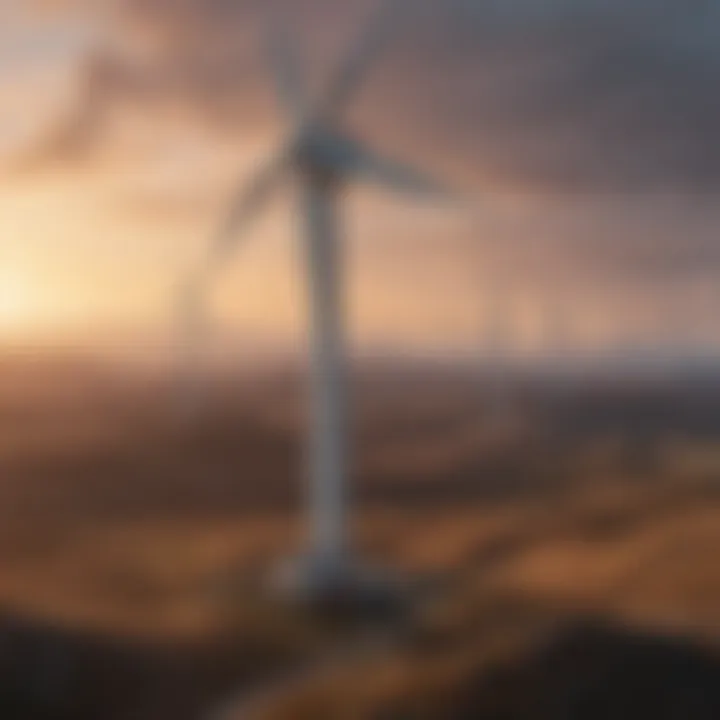
- The key characteristic of zoning laws is that they vary widely between regions, impacting where turbines can be placed.
- The unique feature of these laws is how they can restrict turbine placements based on local opposition or land use priorities. Such conditions complicate the planning phase for developers.
Environmental Regulations
Environmental regulations are necessary for ensuring that wind turbine deployments are conducted responsibly. Compliance with these regulations can affect project timelines and costs.
- The key characteristic of environmental regulations is their critical role in protecting local ecosystems, which can impact decision-making.
- A unique feature of these regulations is that they require thorough assessments and studies which can add to the complexity and duration of the project review process.
Addressing both economic barriers and regulatory constraints is necessary for maximizing the integration of high output wind turbines into the global energy framework. Understanding these challenges is critical for stakeholders to craft effective strategies moving forward.
Environmental Impact
The environmental impact of high output wind turbines is a critical focus due to their growing role in renewable energy solutions. Understanding these impacts is essential for assessing the sustainability and viability of wind energy as a clean power source. This section aims to investigate various ecological and life cycle factors, all contributing to informed decision-making and ongoing enhancements in wind turbine technology.
Ecological Considerations
Bird and Bat Interaction Studies
Studies on bird and bat interactions with wind turbines are important in evaluating the ecological cost of deploying these energy systems. Such studies assess collision risks that birds and bats face when in proximity to turbine installations. Key characteristics of these studies include their reliance on observational data and technological advancements that aid in monitoring wildlife movements. These studies help in understanding deterrent measures and turbine designs that can minimize bird and bat fatalities. However, a unique feature is the complexity of wildlife behavior, which can make it difficult to predict interactions. These studies serve to illuminate important conservation considerations, though they often face criticism for their high variability in outcomes.
Habitat Disruption Assessments
Habitat disruption assessments involve evaluating how the installation of wind turbines impacts local ecosystems. They analyze the direct and indirect effects on flora and fauna in the vicinity. The key characteristic of these assessments is their systematic nature, often employing pre- and post-installation comparisons. Understanding habitat disruption is a beneficial choice for this article, as it highlights the need for environmental mitigation measures. One unique feature to note is the potential for long-term ecological recovery, depending on the restoration efforts post-deployment. Officers and planners must balance energy generation needs against preserving biodiversity.
Noise Pollution Effects
Noise pollution from wind turbines is another area of ecological concern. This form of pollution stems from turbine operation, which can affect nearby wildlife and human communities. The key characteristics of studying noise pollution effects include measuring decibel levels and understanding perception differences between species. This makes noise pollution assessment a relevant aspect of evaluating overall environmental effects. Moreover, it raises questions about unique features of turbine placement and acoustics. High sound levels may lead to behavioral changes in wildlife, which is a significant drawback in terms of operational siting.
Life Cycle Analysis
Life cycle analysis of wind turbines evaluates their environmental impact from production through to decommissioning. This comprehensive approach sheds light on energy investments and waste factors throughout the turbine's life. Understanding the life cycle provides insight into optimizing design and reducing the environmental footprint at each phase.
Carbon Footprint Evaluation
Carbon footprint evaluation focuses on quantifying greenhouse gas emissions across the wind turbine's life cycle. This analysis begins with raw material extraction and proceeds through manufacturing, transportation, installation, and end-of-life management. The key characteristic of carbon footprint evaluation is its ability to provide a clear picture of the turbine’s overall contribution to climate change mitigation. This analysis is a beneficial choice for this article since reducing the carbon footprint aligns with global sustainability goals. A unique feature is the identification of critical stages where emissions can be minimized, ensuring that wind energy becomes a more efficient alternative.
Recycling and End-of-Life Management
The recycling and end-of-life management of wind turbines are critical to ensuring persistent sustainability. This topic revolves around the processes for decommissioning turbines and handling materials, like fiberglass and metals. A key characteristic includes examining economic feasibility and technology to reuse materials. This subject is a beneficial choice because it advocates for circular economy principles within renewable energy. The unique feature worth noting is the increasing innovation in recycling methods, although they still face challenges in addressing composite materials used in blades. These efforts are essential for reducing landfill waste.
Sustainability Benchmarks
Sustainability benchmarks provide criteria for evaluating the environmental performance of wind turbines over time. These benchmarks help to establish best practices in the industry. The key characteristic is their ability to quantify parameters such as energy efficiency, carbon neutrality, and material sustainability. Inclusion of these benchmarks is a beneficial choice for this article, as they guide stakeholders toward enhanced operational standards. A unique feature is that benchmarks allow for comparative analysis among different energy sources, showcasing the relative advantages of wind energy. Setting rigorous benchmarks remains vital for fostering continued improvement in sustainability.
Future Directions
The landscape of high output wind turbines is rapidly evolving. Future Directions in this field are critical for enhancing efficiency, usability, and sustainability. These advancements not only promise to improve current technologies but also pave the way for increased deployment across diverse environments. As society moves towards cleaner energy solutions, understanding these future trajectories becomes essential. The continuous investment in research and development reflects the significance placed on these innovations.
Emerging Technologies
Artificial Intelligence in Wind Operations
Artificial Intelligence has become a key player in optimizing wind turbine performance. Its ability to analyze massive datasets in real-time enhances operational efficiency. For instance, algorithms can predict maintenance needs, reducing unplanned outages. This anticipatory capability is a strong point of AI's application, making it a popular choice in the energy sector. However, reliance on AI for decision-making may pose risks, especially in the event of system failures. It is important to maintain a balance between machine decision-making and human oversight.
Advanced Materials for Increased Efficiency
The development of advanced materials significantly boosts the efficiency of wind turbines. These materials are designed to be lighter, yet stronger, allowing for larger blades without the usual structural limitations. This characteristic contributes to increased energy capture. The innovative composites used can also enhance durability, reducing wear and tear. However, the environmental impact of sourcing these materials must be considered. Thus, while advanced materials bring improvements, their production and lifecycle need thorough evaluation.
Floating Wind Turbine Concepts
Floating wind turbines represent a revolutionary advancement in offshore wind energy. These structures can be placed in deeper waters where winds are stronger and more consistent. Their stability on the water is a significant feature, enabling the harnessing of previously untapped resources. Floating concepts can accommodate larger turbine blades, further maximizing output potential. Nonetheless, challenges exist in terms of installation and maintenance costs. Despite these issues, they hold promise for expanding the reach of wind energy production.
Global Market Trends
Market Projections
Market projections indicate a robust growth trajectory for high output wind turbines. Analysts predict a doubling of installed capacity over the next decade. This growth is driven by rising demand for renewable energy and advancements in turbine technology. It demonstrates the sector's resilience and its adaptation to market needs. However, fluctuations in fossil fuel prices and geopolitical factors can influence these projections. Thus, market conditions will play a pivotal role in shaping the future of wind power.
Investment Trends
Investment trends signal increasing interest in renewable energy projects, particularly high output wind turbines. Financial backing from both private and public sectors is essential for research and deployment. Enhanced return on investment models are being developed to attract investors. Projects aimed at scaling up technology often secure funding due to their potential high impact. However, uncertain regulatory frameworks can deter investment, making a stable policy environment crucial.
Impact of Policy Changes
The impact of policy changes cannot be understated in the wind energy sector. Government incentives and changes in regulations can either facilitate or hinder turbine deployment. Strong policies can create favorable conditions for investment, while abrupt changes can lead to market hesitancy. Additionally, policies that promote cleaner energy measures can accelerate the transition to high output wind technologies. Stakeholders must remain adaptable to navigate the shifting landscape of energy regulation, ensuring continued advancement in this crucial field.
End
The examination of high output wind turbines reveals their significant role in the renewable energy landscape. Incorporating the latest advancements in technology, these turbines stand as a pillar for efficient energy production, directly addressing global energy demands. The focus on both the applications and the implications of deploying such turbines illustrates their critical contribution to sustainable energy solutions.
Summary of Key Points
- Technological Advancements: The incorporation of artificial intelligence and advanced materials enhances operational efficiency and expands the potential for various installation types.
- Applications: High output wind turbines are not limited to large-scale projects; they are flexible and adaptable for use in both onshore and offshore settings.
- Environmental Impact: While these turbines provide clean energy, careful assessment of ecological repercussions is necessary to balance their deployment and environmental safety.
- Economic Factors: Addressing initial investment costs and understanding return on investment are crucial for promoting wider adoption of high output wind turbines.
- Future Directions: Keeping an eye on market trends and policy changes will be vital for stakeholders and investors in the renewable energy sector.
Call for Further Research
The need for deeper inquiry into various aspects of high output wind turbines is evident.
- More studies on bird and bat interactions can help mitigate ecological impacts, allowing for the design of environmentally safer turbines.
- Life cycle analyses are necessary to understand the true sustainability of materials used in turbine production.
- Research into hybrid energy systems that combine wind efficiency with other renewable sources would pave the way for innovative energy solutions.
- Engaging with economic models can provide insights on scaling investments and identifying potential funding avenues for future projects.