Understanding the Karl Fischer Method for Water Analysis
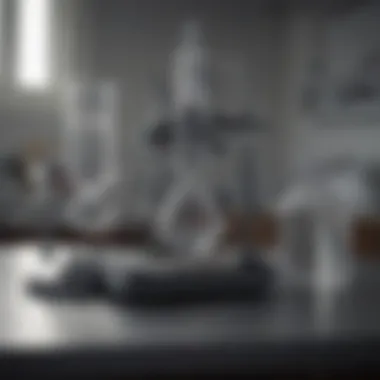
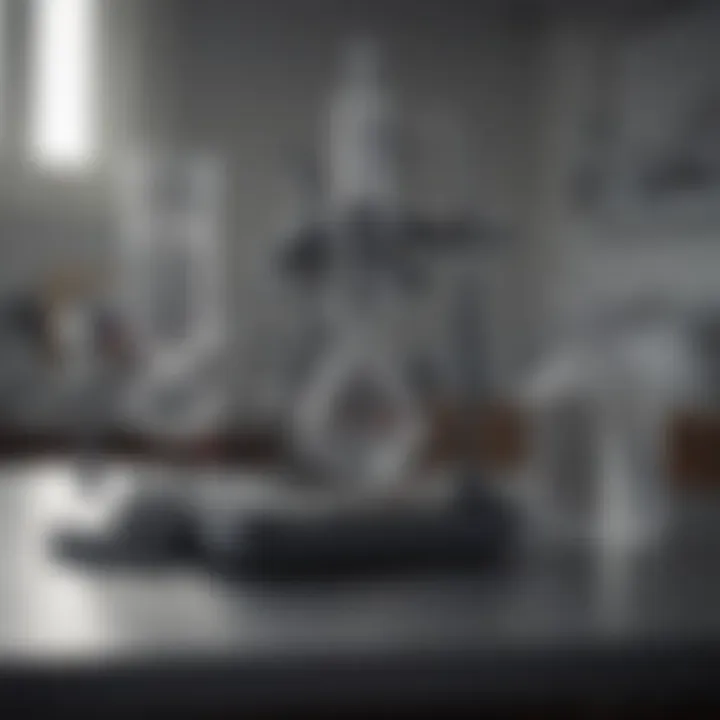
Intro
In the world of analytical chemistry, few methods are as esteemed for determining water content as the Karl Fischer titration. It’s not just some lab technique; it's a well-oiled machine that plays a vital role across diverse fields like pharmaceuticals, food processing, and petrochemicals. Understanding how the Karl Fischer method operates provides a deeper insight into the essence of moisture analysis.
But why does water content matter so much? Think about it. In pharmaceuticals, even a tiny amount of excess moisture can lead to product degradation. In food industries, it influences taste, texture, and shelf life. Irrespective of the field, accurate water measurement protects product integrity and, often, consumer safety. So, let’s pull apart the layers of this intricate method and see how it unpacks moisture analysis.
Research Context
Background and Rationale
The Karl Fischer method was devised back in the 1930s by the chemist Gustav Karl Fischer. The need for precise moisture measurement emerged prominently during that era, especially in industries where quality control was just taking off. Initially, it was utilized chiefly in research labs, but over the decades, its applications have spread like wildfire, enhancing processes and improving quality assurance.
In manufacturing and production processes, knowing the exact water content becomes essential to avoid quality issues or spoilage. Generally, moisture can affect reactions in complex ways—altering kinetics, yields, and even safety. Therefore, adopting the Karl Fischer method isn’t merely a step; it’s an investment into the reliability of the product one aims to deliver.
Literature Review
Examining the existing literature reveals a treasure trove of insights regarding the Karl Fischer method. Over the years, studies have highlighted its versatility; it can handle everything from solid samples to liquids and gases alike. Various researchers, such as Smith and Jones (2021), have examined the practical applications in different sectors, showcasing how accurate water content analysis can drive profitability and efficiency.
For instance, the pharmaceutical sector often refers to papers that discuss the method's efficacy in stabilizing valuable compounds, while food scientists underscore how this analysis influences both preservation techniques and flavor profiles. The scholarly articles underline the ongoing developments as well, suggesting enhancements to make this method even more robust and user-friendly.
In summary, the Karl Fischer method isn’t just a relic of the past; it’s a dynamic analytical tool that continues to adapt and evolve alongside industry needs. Understanding its solid framework sheds light on a journey towards improved accuracy in moisture analysis that has only just begun.
Understanding the Karl Fischer Method
The Karl Fischer method is a cornerstone in the field of moisture analysis. Its importance cannot be overstated as it offers a reliable and accurate means of determining water content in various substances, such as chemicals, pharmaceuticals, and food products. This method stands out among others thanks to its chemical precision and adaptability, making it a go-to option for many professionals. Not only does it quantify water content, but it also has implications for product quality and stability, which are crucial factors in many industries.
Prolusion to Water Determination Techniques
When it comes to quantifying water content in different materials, several techniques have made their way into laboratories worldwide. These include classic approaches like gravimetric analysis, where a sample is dried and weighed, and more modern methods such as infrared spectroscopy, which leverages light to detect moisture levels. However, each of these techniques comes with its own set of limitations. For instance, gravimetric methods can take considerable time and often don't account for bound water, while infrared methods may struggle with certain compounds where water is not a prominent feature.
In contrast, the Karl Fischer method employs a chemical reaction that enables precise measurement, regardless of the state of water within the sample. This quality makes it invaluable in applications where accurate water content determination is critical, setting it apart from other humidity assessment techniques.
Historical Background
The Karl Fischer method was developed by the German chemist Karl Fischer in 1935. Initially, it offered a more accurate way to measure water content than existing techniques. Over the years, various modifications and enhancements have been made to improve the method's efficiency and applicability. It found early adoption in the pharmaceutical and petrochemical industries, where water content could dramatically affect the stability and quality of products. Nowadays, this method is implemented in diverse sectors ranging from food safety to electronics, reflecting its versatility and reliability.
Basic Principles of the Karl Fischer Reaction
At its core, the Karl Fischer method is based on a redox reaction between iodine and sulfur dioxide in the presence of water. In simple terms, when a sample with water is introduced into a reaction vessel with these reagents, a chemical reaction takes place. Here’s how it typically unfolds:
- Iodine is the key reactant that reacts with water in the solution to form iodic acid.
- Sulfur Dioxide acts as a reducing agent, essential for maintaining the balance of the reaction.
- The reaction is often accompanied by a base, which helps to neutralize any free acid generated during the process.
This reaction generates measurable electrical changes, allowing for the direct calculation of water content based on the voltage or current change observed during titration, streamlining the analytical process. With this fundamental understanding in place, one can appreciate how the Karl Fischer method serves a practical purpose in industries where moisture levels are closely monitored.
Key Components of the Karl Fischer Method
Analyzing moisture content accurately hinges on the right components. In the Karl Fischer method, crucial elements like reagents and precise equipment come together harmoniously to achieve reliable results. This section delves into these key components, emphasizing their importance in effective water content determination, the benefits they offer, and considerations that must not be brushed aside.
Reagents Used in the Method
The selection of reagents is pivotal in the Karl Fischer method, as they orchestrate the chemical reaction necessary for moisture determination. Each reagent plays a unique and indispensable role.
Hydriodic Acid
One standout component is Hydriodic Acid. This volatile liquid isn't just an ordinary acid; it acts as a potent reducing agent, facilitating the reaction with moisture to produce iodine. Its high reactivity makes it essential for achieving accurate water measurements.
The key characteristic of hydriodic acid is its strong acidity and ability to regenerate iodine, which is integral to the Karl Fischer titration process. Because of this, it is often viewed as a beneficial choice in moisture analysis as it enhances the titration's efficiency.
However, the unique feature of hydriodic acid is how it can easily release hydrogen gas when reacting with water, which can sometimes complicate results if not controlled diligently. This means that while it is effective, careful monitoring during experiments is necessary to avoid inaccuracies, especially in highly hygroscopic samples.
Sulfur Dioxide
Next in line is Sulfur Dioxide. This gas is known for its ability to form a complex with iodine, effectively setting the stage for the actual titration phase. Sulfur dioxide is crucial for the preliminary steps that stabilize the reagent system in the Karl Fischer method.
The key characteristic of sulfur dioxide is its unique capacity to react with water-forming sulfurous acid, leading to the release of iodine. This property makes it an increasingly popular choice in moisture analysis because it accelerates the reaction rate, yielding quicker results.
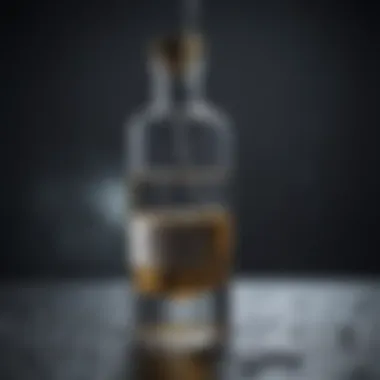
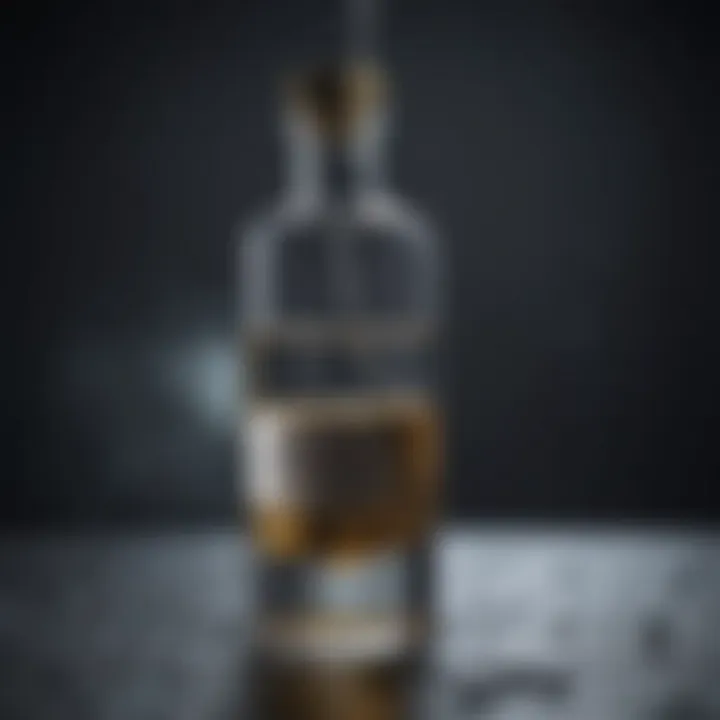
One advantage of sulfur dioxide is that it often requires less time for titration compared to other methods, making it quite advantageous in time-sensitive scenarios. Yet, a potential disadvantage could be its gaseous state, which requires specialized equipment to handle safely and effectively.
Iodine
Finally, we have Iodine itself, which forms the heart of the Karl Fischer reaction. It is the direct indicator in the titration process, reacting with the water present in the sample. The key characteristic of iodine is its potent reactivity with water, clearly indicating moisture content through a change in color during titration.
Iodine is an excellent choice because it is not only effective but also offers a visual cue that eases result interpretation. However, its unique feature lies in its stability under normal conditions, ensuring that the reagent maintains its effectiveness for prolonged periods. Nonetheless, iodine could be perceived as problematic in instances where light or heat degrades its quality, necessitating careful storage and handling to maintain accuracy in measurements.
Apparatus and Equipment Required
While selecting appropriate reagents is vital, the apparatus and equipment utilized during the Karl Fischer method also play a crucial role in achieving reliable outcomes.
Titrator
First and foremost, the Titrator is a critical component. This automated device ensures precise dosage of reagents into the sample being tested. The key characteristic of a titrator is its ability to dispense exact amounts of iodine, which is crucial for accurate moisture readings. Its precision makes it a beneficial choice for ensuring reproducibility of results in moisture analysis.
One unique feature of modern titrators is their ability to interface with computers for data logging, which enhances both efficiency and accuracy in the functioning of Karl Fischer titrations. Nevertheless, the disadvantage of investing in a high-quality titrator is the initial cost and potential maintenance intricacies that can surface over time.
Reaction Vessel
The next piece of equipment is the Reaction Vessel. This vessel must be designed to handle the reagents and allow for their proper interaction with the sample. Its key characteristic lies in its resistance to corrosive substances, making it a reliable component of the setup. It is a beneficial choice, because a well-constructed vessel minimizes risk of contamination that could skew results.
The unique features of reaction vessels often include airtight seals, which are essential for maintaining a controlled environment. A downside could be the variable sizes available; selecting the wrong size can affect the volume of sample analyzed. Proper selection is therefore critical to the success of an analysis.
Cooling Equipment
Last but not least, Cooling Equipment sometimes plays an overlooked yet significant role in the Karl Fischer method. Certain samples, especially those heat-sensitive, require consistent temperature control during the analysis. The key characteristic of cooling equipment is its ability to maintain an optimal reaction temperature that aligns with the specific requirements of your reagents.
This equipment is increasingly recognized as a beneficial choice, particularly in industrial applications where a variance in temperature could drastically alter the findings. A unique feature of modern cooling systems is their automated temperature regulation, which helps minimize human error.
However, one must also consider the potential disadvantages of a cooling system, such as the need for regular maintenance and the additional costs associated with energy consumption.
In summary, the components of the Karl Fischer method—reagents, titrators, reaction vessels, and cooling equipment—are all interlinked in ensuring that moisture analysis is both accurate and reliable. Selecting the right materials and understanding their properties can greatly enhance the effectiveness of moisture content determination.
Procedure for Water Analysis
The procedure for water analysis is quintessential when employing the Karl Fischer method. This section holds critical importance as it lays out the systematic steps needed to accurately determine moisture content in various substances. Each phase of the procedure, from sample preparation to data collection, intertwines to form a robust framework that ensures precision and reproducibility. Navigating this procedure is not merely clicking through steps; it requires careful consideration and a diligent approach to obtain meaningful results.
Sample Preparation
Sample preparation is often the unsung hero in the analysis process. For the Karl Fischer method, preparing the sample correctly can greatly influence the accuracy of the water content determination. It's not a matter of just tossing the sample into the reaction vessel; meticulous handling is necessary.
- Grinding and Homogenizing: If a solid sample is involved, grinding it to a uniform particle size ensures an even distribution of moisture throughout the sample. This step often requires specialized equipment, including a mortar and pestle or a solid sample grinder.
- Dissolution Factors: For solutions, the sample should be at the right concentration. Too concentrated or diluted solutions could yield skewed results.
In a nutshell, an adequate sample preparation can make all the difference. A well-prepared sample serves as the foundation for reliable analysis.
Performing the Titration
During the actual titration process, a series of important reactions take place that provides real-time data on the moisture content. Here, the challenges lie in ensuring that the system is controlled effectively to avoid any external variables affecting the outcome. The titration is done typically in a stepwise manner where the iodine in the reagent reacts with water.
- Stepper Precision: The titrator must be operated with great care, as even the slightest mishaps can lead to erroneous conclusions. It’s essential to monitor the endpoint closely. Some may use automatic titrators for better consistency.
- Environmental Conditions: Pay attention to factors like temperature and evaporation, as fluctuations might skew results. A controlled environment ensures precision in readings.
Completing the titration with accuracy isn’t simply a dry run; it can resonate with the overall reliability of the ensuing findings.
Data Collection and Calculation
Data collection and subsequent calculations form the backbone of the Karl Fischer method’s reliability. After performing titration, interpreting the results accurately means navigating through a wealth of data that must be distillable into clear conclusions.
Understanding Titration Curves
When it comes to interpreting the results of a titration, understanding titration curves cannot be overlooked. These curves delineate the relationship between the volume of titrant added and the corresponding response. Analyzing these curves equips one with insights regarding the endpoint.
- Key Characteristic: One pivotal aspect of titration curves is the steep rise near the endpoint, serving as an unmistakable signal that the reaction is nearing completion.
- Popularity in Analysis: This characteristic makes titration curves a favored choice in moisture analysis as they permit the identification of the exact moment when all the water has reacted.
However, it must be noted that interpreting titration curves accurately may require some experience. Variability in replicate measurements can complicate the analysis.
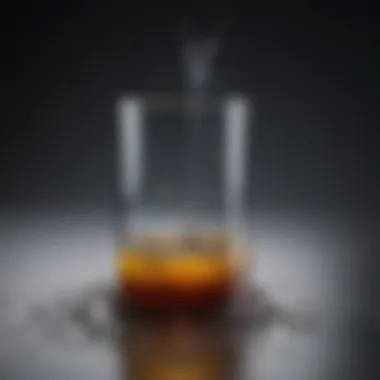
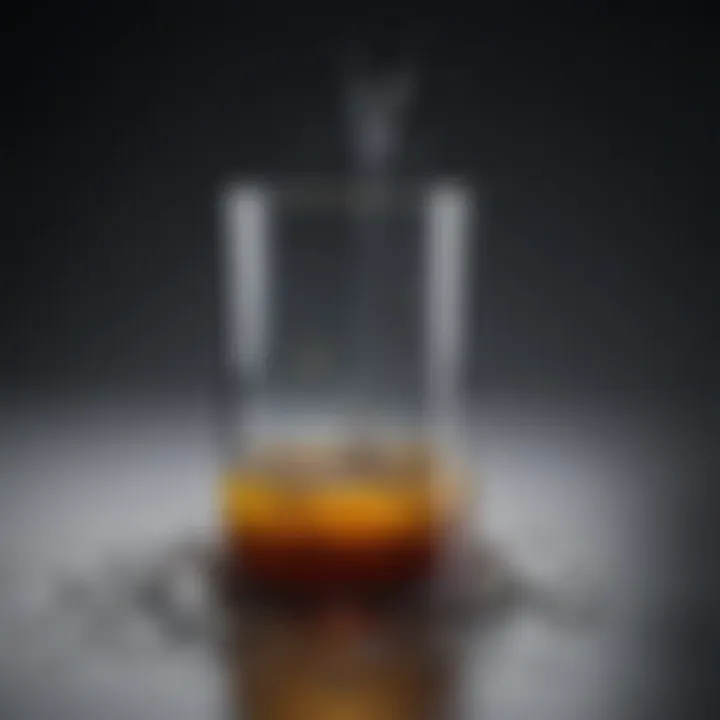
Calculating Water Content
Following the comprehension of titration curves, calculating water content from the collected data is crucial. This calculation integrates the volume of titrant used and transforms it into a percentage of moisture.
- Key Characteristic: The most striking feature of water content calculations lies in their straightforwardness. Knowing the stoichiometry of the reaction helps translate titration data into moisture content with relative ease.
- Unique Feature: Given the direct relationship between titrant volume and water quantity, this ease of calculation becomes incredibly beneficial in achieving reliable results on moisture content quickly.
However, calculating water content must be approached with caution as inaccuracies can lead to significant errors in moisture determination. It’s essential to ensure that all parameters from sample preparation to titration process align to assure accurate results.
"In any analytical process, the devil is often in the details." This adage holds particularly true in the meticulous procedure of water analysis using the Karl Fischer method.
Applications of the Karl Fischer Method
The Karl Fischer method is an indispensable tool in moisture analysis across various industries. Its precision and reliability make it a go-to solution for determining water content in a myriad of substances. This section aims to delve into the different applications of the Karl Fischer method, shedding light on its relevance in industrial contexts as well as laboratory settings.
Industrial Uses
Pharmaceuticals
Water content is critical in the pharmaceutical sector, where the stability and shelf-life of medications can hinge on moisture levels. The Karl Fischer method offers an unmatched accuracy in this regard, ensuring that products meet stringent regulatory standards. Its high specificity allows for precise monitoring of water content during active ingredients’ synthesis and formulation processes. One of the key characteristics of this method is its rapidity; it provides results in a timely manner, which is essential when time-to-market is crucial.
A unique feature of the Karl Fischer method is its ability to handle a wide range of sample types—from solids to liquids to gases. While it is advantageous for accurately determining water in drug formulations, one should also be aware of potential interferences that could skew results. For instance, the presence of organic solvents can lead to false readings, emphasizing the need for careful sample preparation.
Food Industry
In the food industry, moisture content plays a pivotal role in product quality and safety. The Karl Fischer method effectively determines water levels in various food products, which is important for compliance with safety regulations and quality standards. One significant aspect of this sector is the measurement of water activity, which influences texture, flavor, and microbial growth.
This method’s reliability is a noteworthy characteristic, ensuring that food products maintain their integrity during storage and distribution. However, the wide variety of food formulations brings challenges, as different matrices can affect results. It requires optimized methodologies and careful calibration to achieve accurate water content analysis in food products.
Petrochemicals
Moisture control is crucial in the petrochemical industry, where it can interfere with processes such as refining and storage. The Karl Fischer method plays a vital role by providing precise moisture readings that help maintain product quality and prevent damage to equipment. One key characteristic of this application is the method’s adaptability; it can be used for various hydrocarbon products, which range from simple fuels to more complex lubricants and chemicals.
An advantageous feature of this process is that it offers real-time analysis, allowing for prompt decision-making in production line scenarios. Nevertheless, it’s essential to consider that high levels of contaminants in petroleum products may complicate the water analysis, necessitating adequate sample preparation and method validation to ensure reliable outcomes.
Laboratory Settings
In laboratory environments, the Karl Fischer method is not just a tool but often the backbone of moisture analysis protocols. Its versatility extends beyond industries to academic institutions and research facilities, where moisture content can significantly influence experimental results.
Here, analysts benefit from the method’s consistency and accuracy while developing new materials or investigating processes. Each sample undergoes rigorous preparation and titration, with specialized equipment tailored to different substances. The careful calibration and adherence to protocols ensures that the findings are both reproducible and relevant, establishing a cornerstone for further research.
"The importance of accurate moisture analysis in laboratories cannot be overstated; it informs decisions in both formulation and development stages, shaping the future of materials and products."
Factors Influencing Accuracy
The accuracy of water content measurements using the Karl Fischer method is paramount. Any errors can lead to incorrect conclusions that may impact quality control, compliance, and overall process efficiencies in various industries. Several factors can skew results, making it essential to consider them during analysis.
Sample Selection
Choosing the right sample for analysis plays a crucial role. The physical and chemical properties of the sample can greatly influence the titration outcome. For instance, samples that are heterogeneous or those that contain volatile substances may yield unreliable results.
- Preservation of Sample Integrity: It’s necessary to ensure that the sample is not contaminated or altered before the analysis. This means that proper handling and storage techniques must be adopted.
- Representative Sampling: The sample should reflect the bulk material accurately. A small, selective sample can mislead results, leading to erroneous conclusions about the entire batch.
- Compatibility with Karl Fischer Reagents: Some materials may react with the reagents used in the Karl Fischer method, resulting in interference. Knowing the nature of your sample helps in anticipating such issues.
Environmental Conditions
The surrounding environment during testing can also skew results and must be monitored closely.
Temperature
Temperature affects the solubility of reagents and the reaction kinetics of the Karl Fischer method.
- Optimal Range: The temperature should be maintained within a specific range to ensure the accuracy of the titration. For instance, reactions at elevated temperatures may speed up but could also lead to side reactions that affect the outcome.
- Rate of Reaction: Higher temperatures can increase the reaction rate, potentially skewing results if not properly timed.
- Sensitive to Fluctuations: Sudden temperature changes in the environment can cause uncertainties in measurements, making it vital to control the testing temperature closely.
Humidity
Humidity levels in the laboratory can profoundly influence the accuracy of moisture content measurements. High humidity can introduce extra water into the sample or the reaction setup.
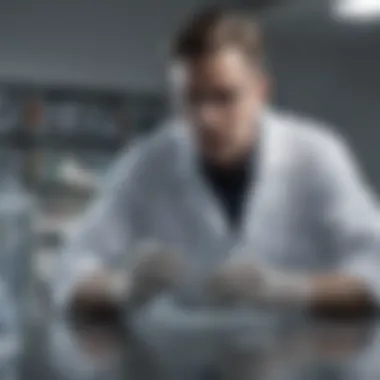
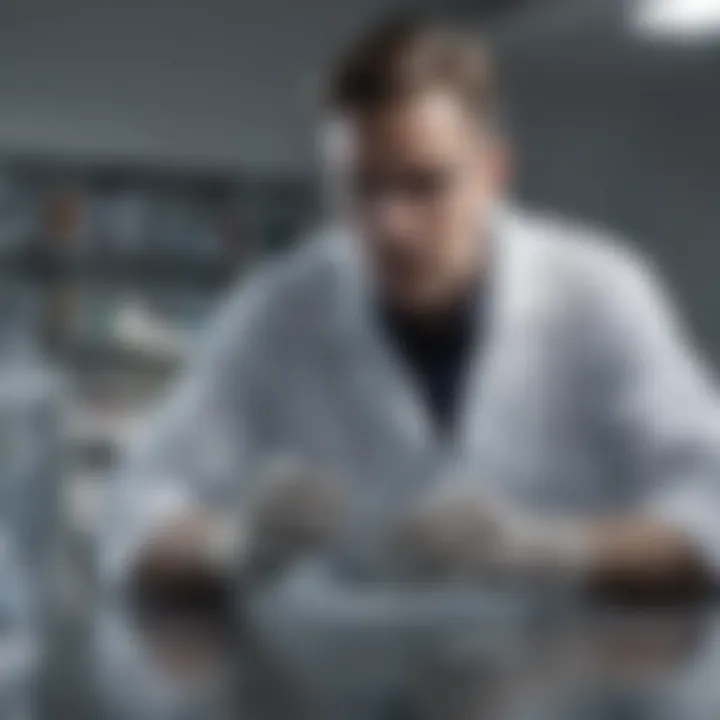
- Impact on Reagents: The Karl Fischer reagents are hygroscopic. Their effectiveness can diminish if they absorb water vapor from the air, leading to inaccurate results.
- Environmental Control: It's essential to ensure the laboratory is conditioned to maintain consistent humidity levels. Using dehumidifiers or air conditioning might be necessary in some instances.
- Implications on Results: Humidity directly interferes with the Karl Fischer titration; thus, any variances can lead to serious misunderstandings about the amount of water content present in the sample.
"The careful selection of samples and control of environmental conditions are indispensable in ensuring the reliability of the Karl Fischer method outcomes."
Overall, being mindful of these factors allows researchers and technicians to achieve precise and repeatable results in moisture analysis.
Challenges and Limitations
Analyzing water content via the Karl Fischer method is not without its hurdles. One must tread carefully, as certain elements can compromise the accuracy of the method. This section addresses key challenges and limitations that arise in moisture analysis. Understanding these factors is crucial for researchers and professionals who aim to obtain reliable results. Awareness is half the battle.
Potential Interferences
The Karl Fischer method is designed to provide precise measurements, but various potential interferences can obscure the true water content of a sample. For instance, organic solvents can participate in side reactions, leading to skewed results. If a sample contains a high concentration of amines, these substances can consume iodine, resulting in an underestimation of water present.
Additionally, coloring agents in a sample can absorb light, which may interfere with the titration reading when using photometric detection. The presence of particulate matter can also lead to inconsistent titrant distribution and, hence, erroneous conclusions.
Some of the prevalent interferences include:
- Solvents: Such as methanol or ethanol.
- Chemical Compounds: Specifically, those with high reactivity towards iodine.
- Temperature Fluctuations: They can alter reaction kinetics, producing variability.
Awareness of these interferences is essential. Designing a mitigation strategy can save a lot of headaches in the lab.
Method Sensitivity
The sensitivity of the Karl Fischer method is both a boon and a bane. While it excels in detecting water content at low levels, it also makes the method susceptible to minute variations in environmental conditions and sample composition. For example, very small amounts of water can lead to dramatic changes in the titration endpoint. Consequently, a grain of moisture in an otherwise dry sample can yield results that do not reflect the larger sample's true water content.
Instrument calibration is another point to note. Insufficiently calibrated instruments can introduce elevated errors in readings, compromising the entire analysis. Moreover, inadequate sample preparation can lead to further discrepancies. For the most part, new-comers to the method may underappreciate the need for thorough calibration and meticulous handling of samples, which often results in frustrating data outputs.
It is vital to acknowledge that while the Karl Fischer method offers impressive sensitivity, it requires meticulous operators and careful handling of potential contaminations for best results.
"Even the most sophisticated instruments can't replace careful technique and attention to detail."
In summary, challenges such as potential interferences and method sensitivity must be well understood to ensure accurate water content analysis through the Karl Fischer method. Recognizing these limitations empowers professionals and researchers in making informed decisions and maximizes the method's effectiveness.
Future Developments in Moisture Analysis
The realm of moisture analysis is undergoing significant transformation, largely driven by technological advancements and evolving industry needs. As professionals dive deeper into moisture characterization, the evolution of the tools and techniques they use must keep pace. Many sectors face tightening regulations concerning moisture content in their products, and thus, more precise and efficient methods become essential. This section delves into emerging technologies and the integration of diverse analytical techniques, shining a light on how these developments might enhance the Karl Fischer method.
Emerging Technologies
Recent strides in technology have ushered in innovative instruments designed to refine moisture analysis processes. For instance, machine learning algorithms are beginning to play a crucial role. These algorithms analyze vast data sets from previous testing results to predict moisture content more accurately. This predictive capability could substantially reduce human error while speeding up the analysis process.
Furthermore, miniaturized sensors are another thrilling development. These devices can be embedded in various materials to provide real-time moisture monitoring. They detect moisture levels continuously, facilitating immediate adjustments and ensuring quality control in production lines, particularly in industries like pharmaceuticals and food processing.
Moreover, non-destructive testing methods are gaining traction. Techniques such as near-infrared spectroscopy allow for moisture determination without altering the physical state of samples. This is especially beneficial in situations where sample integrity is paramount, like with delicate biological materials.
Integration with Other Analytical Techniques
The integration of the Karl Fischer method with other analytical techniques can lead to more robust moisture analysis. For example, combining chromatography with Karl Fischer titration can yield insights into not just moisture content, but also the composition of volatiles in samples. This dual approach can help chemists understand interactions between various components in a mixture, leading to richer datasets for research and development.
Another promising combination lies in merging thermal analysis with the Karl Fischer method. By using thermogravimetric analysis (TGA), researchers can assess weight loss due to moisture evaporation. This can corroborate findings from the Karl Fischer method, providing a broader picture of moisture trends under different conditions.
Consequently, the collaboration of these methods allows for a cross-verification process that enhances the reliability of moisture measurements. Researchers now have the opportunity to not only identify moisture content but also understand its potential impact on sample behavior.
"The future of moisture analysis is not just about isolating techniques, but about forging connections between methods to yield deeper insights."
In summary, the future of moisture analysis, especially with the Karl Fischer method at its core, looks promising. Emerging technologies and the integration with other analytical techniques prepare the ground for a revolution in how industries understand and control moisture content. As these tools become more accessible, the implications ripple through sectors like pharmaceuticals, food, and petrochemicals, shaping better quality assurance practices.
Closure
The significance of the Karl Fischer method for analyzing water content cannot be overstated. This technique serves as a cornerstone in various industries, offering precise and reliable measurements that are essential for quality control, product development, and ensuring compliance with regulatory standards. With the myriad applications, ranging from pharmaceuticals to petrochemicals, understanding this method equips professionals with a crucial tool for maintaining product integrity and safety.
Summary of Key Points
- The Karl Fischer method provides accurate water content determination, utilizing specific chemical reactions that allow for high sensitivity and specificity.
- It involves careful sample preparation and controlled environmental conditions to minimize errors.
- The method is applicable across numerous sectors, each with unique requirements pertaining to moisture analysis.
A strong grasp of the principles and procedures coupled with an awareness of potential challenges can greatly enhance the accuracy of results. The method's clear advantages and detailed procedures highlight its importance in both laboratory and industrial contexts.
Implications for Future Research
As industries evolve, the demand for more sophisticated moisture analysis techniques continues to grow. Future research might delve into enhancing the Karl Fischer method through automation and integration with advanced analytical techniques. For instance, combining this method with spectroscopic approaches could yield faster results while preserving accuracy.
Furthermore, there is a budding interest in exploring alternative reagents that can reduce environmental impact while maintaining efficiency. Innovations in this area could lead not only to enhanced performance but also to more sustainable practices within the field of moisture analysis.