Particle Size Analysis Using Sieve Method
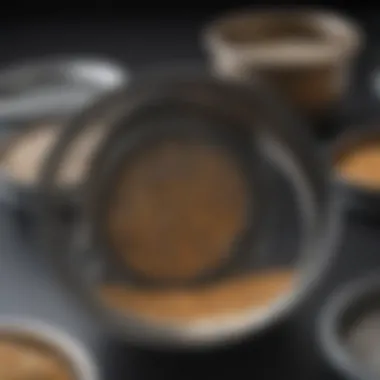
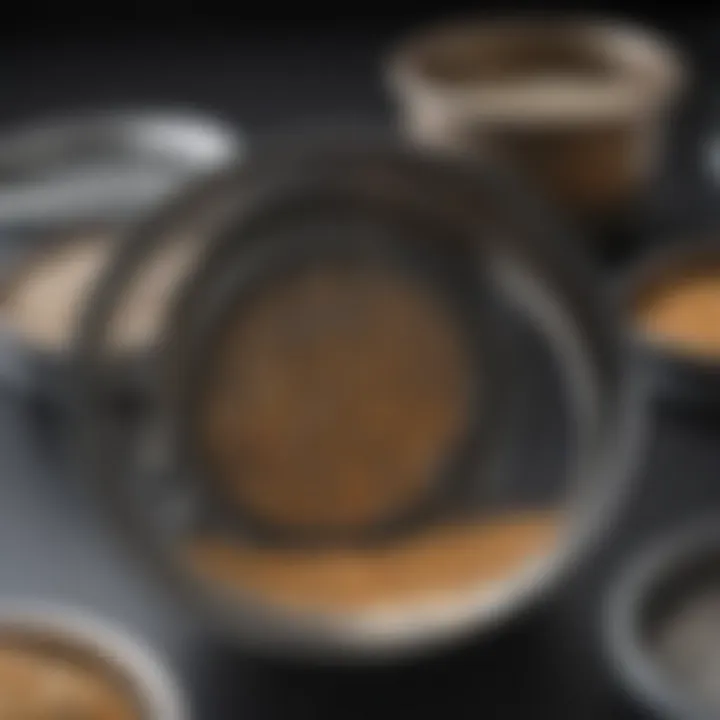
Intro
Understanding particle size is a critical aspect in various fields, from pharmaceuticals to construction and even environmental science. The relationship between particle size and material properties can significantly impact considerations such as flowability, dissolution rates, and overall product stability. One of the most established methods for analyzing particle size is through the sieve method, a technique that has stood the test of time due to its simplicity and reliability.
The sieve method operates on the principle of stratification, where particles are separated based on size through the use of a series of sieves. This method, while straightforward, offers a wealth of information that can inform not only product formulation but also quality control measures. In a sense, it's like peeling back an onion—layer by layer, you uncover insights that are crucial for effective material usage.
As we delve deeper into the sieve method, it’s worth noting the various applications and implications this technique has across diverse disciplines. With an eye on its fundamental principles and methodologies, we can better appreciate its role in shaping efficient industrial practices and advancing scientific research.
"The sieve method not only measures size; it defines how we understand the boundaries of material science.”
In this section, we will explore the research context surrounding the sieve method—first by considering its background and rationale, followed by a review of extensive literature that sheds light on its applications and developments in recent years. These insights will set the stage for further discussions on the methodology employed in particle size analysis using this technique.
Foreword to Particle Size Analysis
Particle size analysis serves as a cornerstone in various scientific fields, underpinning many principles that govern material behavior and functionality. Understanding the size distribution of particles can greatly influence material selection, processing techniques, and ultimately, the performance of the end product. This significance underscores its role in areas such as pharmaceuticals, construction, and environmental science. The sieve method, one of the oldest yet reliable techniques, provides a straightforward and effective means of characterizing particle size.
Importance of Particle Size Characterization
Particle size characterization is critical due to several reasons that stretch across different industries. For one, the size of particles can affect their reactivity, strength, and even dissolution rates in pharmaceutical applications. Consider a scenario in the drug manufacturing process; the efficacy and bioavailability of a medication often hinge on the size of its constituents. Smaller particles tend to dissolve faster than their larger counterparts, leading to quicker absorption into the bloodstream.
In construction materials, particle size can determine the strength and durability of concrete. Coarse aggregates versus fine aggregates both have their specific roles and required particle sizes. If not accurately characterized, the resultant materials could fail to meet design specifications, leading to severe safety concerns.
The natural world also showcases the importance of particle size in environmental contexts. For example, in soil science, the distribution of particle sizes impacts water retention, nutrient availability, and even the biological activity in the ecosystem. Thus, precision in particle size analysis is not merely a technical requirement—it can have broad implications on safety, efficacy, and environmental performance.
Overview of Particle Size Analysis Techniques
Particle size analysis is not confined to just one method; it encompasses a variety of techniques, each with its strengths and weaknesses. Beyond the traditional sieve method, other approaches like laser diffraction and dynamic light scattering (DLS) have gained prominence in particular scenarios. The choice of technique can depend on several factors including sample type, required resolution, and measurement scalability.
- Sieve Analysis: A classic technique that uses a series of sieves with different mesh sizes to separate particles based on size. Simple and cost-effective, it fits well for larger particles commonly found in construction and mineral processing.
- Laser Diffraction: This method is often favored for its ability to measure a wide range of particle sizes rapidly. It operates on the principle of laser light scattered by particles, providing a size distribution profile within moments.
- Dynamic Light Scattering: Primarily used for smaller particles, especially in the nanometer range, DLS measures the Brownian motion of particles to determine their size. This technique excels in applications like colloids or pharmaceutical formulations where high precision is required.
- Microscopy Techniques: Though not exclusively a sizing method, scanning electron microscopy (SEM) or optical microscopy can provide detailed images that allow for accurate particle size determination by counting.
Understanding these various techniques is paramount. The choice of method not only affects the accuracy of the results but also dictates the implications in practical applications. For researchers and professionals, knowing when and how to apply each technique empowers them to harness the full potential of particle size analysis.
Fundamentals of the Sieve Method
Understanding the fundamentals of the sieve method is crucial for anybody looking to excel in particle size analysis. This technique provides not just a means to quantify particle sizes, but it also offers insights into the quality and characteristics of various materials. A firm grasp on the sieve method’s principles empowers researchers and professionals alike to draw meaningful conclusions from their data.
When considering the sieve method, several core elements come to light. First, we have the primary principle that underpins it: the segregation of particles based on size. This sorting process is achieved by utilizing a series of sieves, each with a distinctly sized mesh. The fundamental premise is simple: larger particles remain on top while smaller entities pass through. This physical separation is akin to a filter, ensuring that the collected data reflect the actual distribution of sizes.
In light of its significance, there are multiple benefits associated with employing the sieve method. For starters, it is a cost-effective option when measuring particle size, especially compared to more advanced techniques. The equipment involved is typically straightforward, leading to lower maintenance requirements. Additionally, sieve analysis yields results that can be easily interpreted and integrated into broader data analysis frameworks.
Theoretical Principles Behind Sieve Analysis
At the heart of sieve analysis lies a series of theoretical principles that warrant closer examination. As previously mentioned, the most basic is size segregation. This principle operates on the concept of gravitational settling, where particles descend through a medium—like a mesh—based on their size and weight. The larger particles, unlikely to fit through, remain while the smaller ones fall to the next sieve, creating a stratified collection ultimately reflective of the original sample.
Moreover, it’s critical to consider particle shape and density. These factors can influence how a particle interacts with the sieve. For instance, elongated or irregularly shaped particles may behave differently than spherical ones, potentially affecting the outcomes of the analysis. Testing barefoot in depth in this area can lead to variations in results, where shape could play a significant role in how effectively particles are classified.
Types of Sieves and Their Applications
The types of sieves utilized in analysis can vary widely, each offering unique applications suited to specific materials. To illustrate:
- Standard Test Sieves: Mostly used for traditional analyses, constructed from stainless steel and often used in labs for precise measurements.
- Micro-Mesh Sieves: These are designed for finer materials, perfect for industries focused on very small particle sizes, like pharmaceuticals.
- Heavy-Duty Sieves: Built for rugged environments such as construction, these sieves handle larger, coarser materials and can endure abrasive conditions.
When working with sieves, one must choose appropriately for the task at hand. Not all sieves are created equal; utilizing the wrong one can lead to skewed data. The efficacy of your analysis hinges on selecting the right sieve for your specific application, whether that be in food production or harsh industrial processes.
"The choice of a sieve can be the difference between accurate results and a data nightmare."
In summary, the fundamentals of the sieve method lay the groundwork for a more profound comprehension of particle size analysis. By dissecting both the theoretical principles and the specific types of sieves tailored for various applications, professionals can enhance their approaches to material characterization. Keeping these fundamentals in mind paves the way for more sophisticated experimentation and insight.
Sieve Calibration and Maintenance
In the realm of particle size analysis, particularly when using the sieve method, calibration and maintenance play a pivotal role. These processes not only underpin the accuracy of results but also ensure the reliability of measurements. Regular calibration guarantees that the sieves provide results that are not just close but precisely aligned with the established standards.
Moreover, proper maintenance of sieves prolongs their lifespan and optimizes their performance in various applications, from pharmaceuticals to construction materials. Ignoring these aspects can lead to erroneous results, making clear the necessity of adherence to calibration standards and maintenance routines for achieving credible, high-quality data.
Standard Procedures for Calibration
When discussing calibration, one must consider a structured approach. Standard calibration procedures for sieves typically follow these steps:
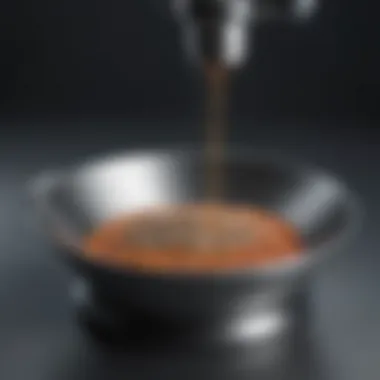
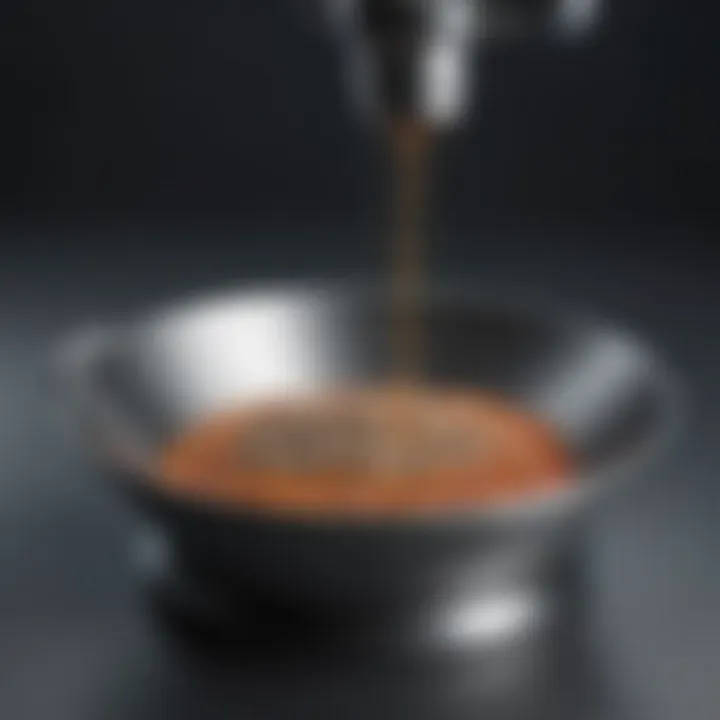
- Selection of Reference Materials: Choose appropriate materials that have known particle sizes to verify the calibration of the sieve.
- Visual Inspection: Conduct a thorough inspection of the sieve mesh for any damages or contaminations. Any discrepancies must be rectified before proceeding.
- Calibration Setup: Using a calibrated balance, weigh a specific amount of the reference material. This weight should typically be representative of normal testing conditions.
- Sieving Process: Agitate the sieve containing the reference material under controlled conditions. It's crucial to ensure consistency during this step, as any variance can skew results.
- Data Recording: After sieving, carefully collect and record the mass fractions. Compare these values against expected outcomes to determine if the sieve maintains its calibration.
- Adjustments: If results deviate significantly from expected values, recalibrate or replace the sieve as necessary.
These steps ensure that each sieve retains its accuracy over time, facilitating credible data collection.
"Calibration is not a one-off task; it's a continuous journey to ensure reliable results in particle size analysis."
Preventive Maintenance Strategies
To guarantee optimal performance and longevity of sieves, establishing preventive maintenance strategies is essential. Here are several effective approaches:
- Regular Cleaning: After each use, clean sieves immediately to avoid residue build-up which can affect subsequent tests. Utilize soft brushes or air blowers for efficacy.
- Routine Inspections: Set a routine for inspecting the sieves for any wear, damage, or deformities. Mesh should be intact, as any tears can allow unwanted particles to pass through.
- Proper Storage: Store sieves in a dry, protected place to prevent exposure to moisture and contaminants. Ensure they are well-organized to avoid any physical damage.
- Training: Provide training for personnel on proper handling and maintenance practices. Improper handling can lead to issues that may compromise the performance of sieves over time.
- Documentation: Maintain detailed records of all calibration and maintenance activities. This documentation can be crucial for audits and enhances traceability in laboratory practices.
Applying these preventive measures will help keep the sieves in peak condition, thereby aiding in consistent and accurate particle size analysis.
Sieve Analysis Procedure
Understanding how to conduct a thorough sieve analysis is central to achieving reliable and comparable results in particle size characterization. This process hinges on precision and accuracy, as even minor missteps during the analysis can skew results and complicate data interpretation. For researchers and industry professionals, grasping the nuances of sieve analysis methods can make a significant difference in the effectiveness and efficiency of material classification in their respective fields.
Sample Preparation Techniques
Before diving into the sieve analysis, the preparation of samples must not be overlooked. Proper sample preparation is fundamental in ensuring that the testing reflects true material characteristics. Here are some steps and considerations:
- Choosing a representative sample:
The first order of business is to pick a sample that accurately represents the bulk material. If you're dealing with a large quantity, take several smaller samples from different spots and mix them well. - Drying the sample:
Moisture can alter the weight of the material, making it lighter than it actually is. Drying the sample ensures that the weight doesn't throw off your analysis. - Breaking up large aggregates:
If your material contains clumps or aggregates, they should be disintegrated prior to sieving. This can typically be achieved with a mortar and pestle or through gentle mechanical means. - Choosing the right amount:
Generally, a sample size of about 100-200 grams is a good rule of thumb for traditional sieve tests. Too large might overload the sieve, while too small can lead to unrepresentative results.
The goals in this phase should focus on reducing variability and maximizing the accuracy of your findings.
Step-by-Step Guide to Sieve Analysis
To navigate through the sieve analysis procedure, having a structured approach is paramount. Here’s a succinct step-by-step guide to lead you through the process:
- Gather necessary equipment:
- Stack the sieves:
- Weigh the sample:
- Commence sieving:
- Evaluate the results:
- A set of sieves with different mesh sizes. Each mesh serves to quantify various particle size ranges.
- A balance for weighing the samples before and after the sieving.
- Appropriate containers or pans to catch the sieved material.
- Arrange the sieves in descending order of mesh size, from top to bottom, with the largest opening at the top and smallest at the bottom. This complements the gravitational force at play during the analysis.
- Accurately record the initial weight of your prepared sample to calculate the distribution of particle sizes afterward.
- Place the sample in the top sieve and securely cover the stack.
- Use a mechanical shaker or manual shaking methods to initiate the sieving process. This will help material path through the sieves over a specified period, usually around 10 to 15 minutes.
- Once the shaking is complete, carefully weigh the contents collected in each sieve. Record these weights for analysis.
- Calculate the percentage of material retained on each sieve by comparing it with the total initial weight. Formulas can be used to derive various metrics such as the cumulative mass distribution.
Thus, precisely following these procedural steps ensures that the data gathered from the sieve analysis is both reliable and valid, permitting a more accurate understanding of your materials' particle size distribution.
In summary, the sieve analysis procedure is a multifaceted approach that requires attention to detail at every step. From adequate sample preparation to systematic analysis, each action intricately ties into the reliability of the final results.
Data Interpretation and Reporting
Data interpretation and reporting play a crucial role in particle size analysis, particularly when utilizing the sieve method. The results derived from sieve analysis are not merely numerical; they convey insights that can significantly influence material selection, processing conditions, and quality control across various industries. Understanding how to accurately interpret these results is paramount for professionals looking to make informed decisions based on particle size distribution.
Accurate analysis allows researchers and industry practitioners to identify trends and variations in material properties. For instance, in the pharmaceutical realm, particle size can affect drug solubility and absorption rates, while in construction, it informs the engineering properties of aggregate materials. Delving into the results requires an appreciation of the methodologies employed during the sieving process, as well as the tools and metrics used for analysis.
One crucial aspect is the representation of results, typically presented as cumulative or differential particle size distribution curves. These graphical representations provide a clear visual interpretation of data, making it easier to draw conclusions and communicate findings effectively. Moreover, an understanding of the statistical parameters such as mean size, variance, and standard deviation can enhance the quality of data reporting, ensuring that all relevant variables are considered.
"Data is not just numbers; it speaks volumes about the material's capabilities and potential applications."
As technology advances, the integration of digital tools for data analysis becomes increasingly important. Software programs can facilitate complex calculations and produce comprehensive reports, minimizing human error and allowing for more sophisticated interpretations, especially relevant in large-scale studies.
Understanding Sieve Analysis Results
The process of interpreting results from sieve analysis hinges on various factors, which include the size of the sieves, the characteristics of the sample material, and the analytical techniques employed. Each sieve contributes to delineating the weight distribution of particles, categorized according to size fractions. For instance, when a sample is passed through a series of sieves, the weight percentage retained is recorded to calculate the cumulative passing on a percentage basis.
Understanding this helps practitioners make sense of how particle size affects the behavior of materials. For example, a higher concentration of finer particles may enhance reactivity in processes such as cement hydration, whereas coarser mixtures could offer increased structural integrity.
Common Reporting Standards
Reporting standards in sieve analysis are governed by various national and international guidelines. Following these standards enhances the credibility of results and facilitates communication across the industry. Commonly referenced standards include the American Society for Testing and Materials (ASTM) specifications, which outline the procedures for conducting sieve analysis and the acceptable limits for sample preparation and results presentation.
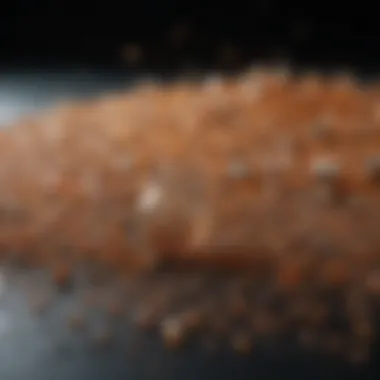
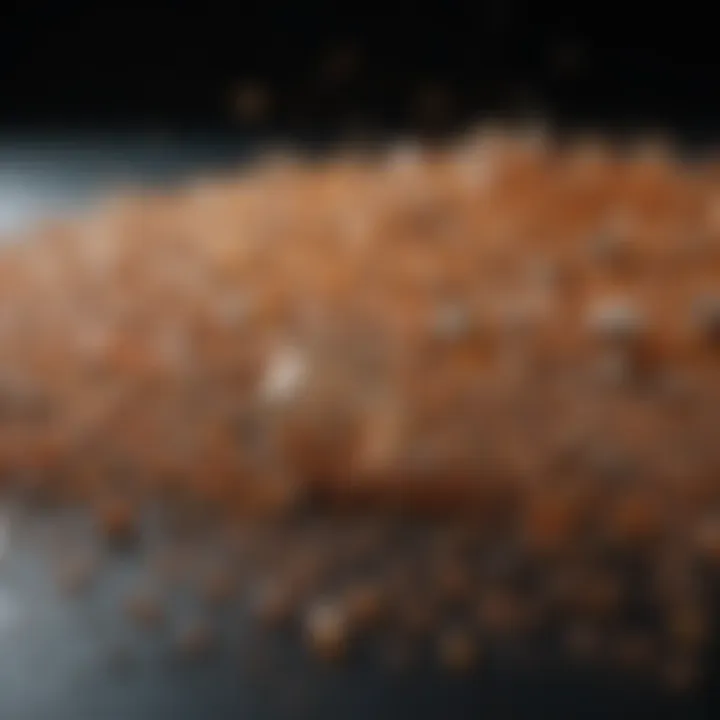
In practice, results should ideally be reported clearly with details such as:
- Sieve mesh sizes used
- Total mass of the sample
- Weight retained on each sieve
- Cumulative weight percentages
- Graphical representations of the particle size distribution
Such structured reporting not only assists in maintaining consistency but also ensures that data is comprehensively captured for subsequent analysis or research. This structured approach can significantly bring clarity, allowing stakeholders to make decisions grounded in verifiable data.
Applications in Various Fields
Particle size analysis using the sieve method serves as a bedrock for numerous industries, impacting product quality and operational efficiency across the board. By providing a systematic approach to characterizing materials, this method lays the groundwork for understanding how size influences various properties, such as flowability, compressibility, and reactivity. In essence, the key insights gained from sieve analysis not only enhance product formulations but also optimize processing methods. Let's examine the implications in three prominent fields:
Pharmaceutical Industry
In the pharmaceutical realm, particle size plays a pivotal role in the bioavailability of drugs. The deposition of fine powders can improve dissolution rates, crucial for patient outcomes. Using the sieve method, manufacturers can guarantee uniformity in size, which directly influences activity levels and consistency in medication.
- Key considerations:
- The regulatory bodies, such as the FDA, emphasize stringent quality control standards where size distribution is critical.
- Smaller particles tend to offer larger surface areas, which can enhance the speed at which therapeutics become active within the body.
Potential challenges include the risk of segregation during handling. If larger and finer particles separate, it can lead to inconsistencies in dosage forms. Hence, accurate sieve analysis can mitigate these risks by ensuring homogeneous mixtures throughout the production process.
Construction and Materials Science
In construction and materials science, the sieve method is essential for determining the size distribution of aggregates used in concrete. The mechanical properties of concrete depend on the characteristics of its constituent materials; thus, a careful analysis of particle size ensures enhanced strength and durability of the final product.
- Impacts of Particle Size:
- Proper grading of aggregates can prevent segregation and ensure better workability of concrete mixtures.
- The sieve method also helps identify the right proportions of fine and coarse aggregates to achieve optimal density.
Moreover, size analysis is utilized in assessing soil composition. For instance, the behavior of soil in construction projects can directly correlate to particle size distribution, influencing stability and compaction characteristics. Ensuring correct sizing through sieve analysis can lead to more reliable and sustainable construction solutions.
Food and Agricultural Products
The food industry heavily relies on particle size analysis to maintain quality control over various products. For example, the texture of flour, the mouthfeel of sauces, and the consistency of pastes all depend on how finely these ingredients have been ground or sifted.
- Significance of Sieve Analysis in Food Products:
- Different applications require specific particle sizes for optimal flavor release and texture stability.
- In the agricultural sector, seed size affects planting density and crop yields, making sieve analysis an important tool in assessing seed quality.
Attention must be paid to hygiene and contamination risks during the analysis, especially in food production settings. Maintaining clean practices and calibrating sieves properly will ensure that the products meet food safety standards.
Understanding the varying demands for particle size across industries highlights the versatility of the sieve method. Its applicability extends beyond mere measurement—it's about embracing a holistic perspective on material interactions and performance.
Challenges and Limitations
When it comes to the sieve method of particle size analysis, understanding the inherent challenges and limitations is crucial. While this technique has served as a reliable method for gauging particle dimensions, it’s not without its flaws. These challenges can complicate data interpretation and overall results, which can affect subsequent applications and conclusions drawn from the analysis.
Limitations of Sieve Analysis
Sieve analysis, despite its widespread use, comes with several limitations that can impact accuracy and reliability. Some of the key downsides include:
- Size Range Constraints: The method is primarily effective for coarse particle sizes. Particles that fall into finer categories often pose a challenge because sieves can’t separate them adequately.
- Non-Sphericity of Particles: Sieve analysis assumes particle shapes are spherical, which is rarely the case in real-world applications. Irregularly shaped particles can yield skewed results, leading to inaccuracies in size distributions.
- Clogging Issues: During analysis, fine particles may clog the sieve, leading to an inability to pass through. This affects the measurement reliability and can result in misleading data.
- Limited Sensitivity: Sieve analysis is not as sensitive as other methods in detecting slight variations in particle size, making it less favorable for applications that demand high precision.
These limitations necessitate a careful approach to interpreting results and incorporating sieve analysis into broader analytical frameworks.
Common Errors in Sieve Analysis
Even seasoned professionals can stumble during particle size analysis using sieves. Recognizing these common errors can save significant time and resources. Here are some pitfalls to watch for:
- Improper Calibration: Failure to regularly calibrate sieves can lead to inaccurate measurements. Regular checks and calibrations are vital for maintaining the effectiveness of the particle size analysis.
- Inadequate Sample Preparation: Pre-analysis procedures play a critical role in the overall accuracy. Failing to appropriately prepare samples can lead to uneven distribution, affecting sieving efficiency.
- Inconsistent Handling: Not following standardized protocols when handling samples and sieves can introduce variability. Each step, from loading samples to recording results, must be handled with precision.
- Ignoring Environmental Factors: Humidity and temperature can impact sieve performance. Failing to control these conditions may lead to variations in particle behavior, skewing results.
"Successful particle size analysis hinges not just on methodology but on a deep understanding of its limitations and potential errors."
Comparative Methods of Particle Size Analysis
Particle size analysis is crucial in many fields, from pharmaceuticals to environmental sciences. While the sieve method holds its own, understanding comparative methods offers a well-rounded view of how particle size can be effectively measured and analyzed. These alternative techniques can highlight various aspects of particle behavior that might be overlooked with sieving alone.
Importance in this Article
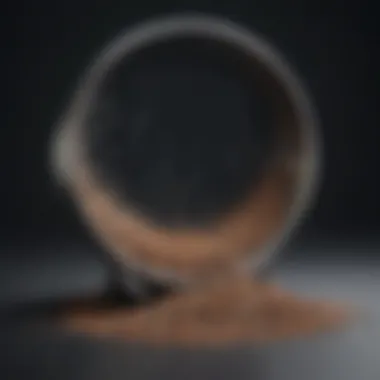
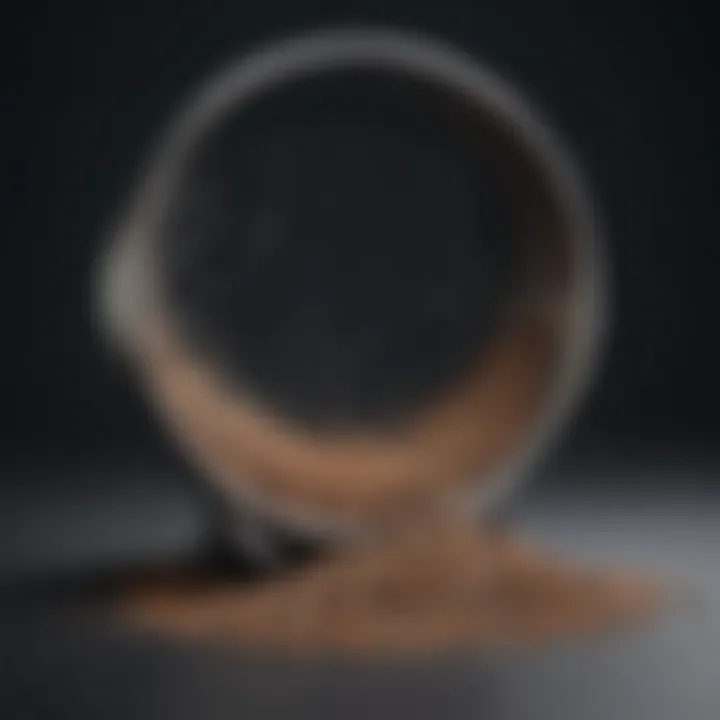
The relevance of examining comparative methods stems from their unique advantages and disadvantages. In many applications, it is not just about size—particle shape, structure, and distribution can play significant roles in determining material performance. This section emphasizes the breadth of techniques available, allowing researchers and practitioners to make informed decisions based on their specific needs.
- Versatility: Different methods can be applied depending on the material and desired particle size range.
- Precision: Alternative techniques may offer more precise measurements or allow for the analysis of finer particles that sieves might miss.
- Complementarity: Using multiple methods can validate findings and provide a more comprehensive analysis.
In summary, exploring these methods not only enhances understanding but also fosters innovation in material characterization.
Laser Diffraction Techniques
Laser diffraction is a sophisticated technique based on the principle that particles, when illuminated by a laser beam, scatter light at angles that correlate with their size. The resulting patterns provide a clear indication of the particle size distribution. Here are some key points about this method:
- Advantages:
- Applications: Frequently employed in industries where particle size significantly impacts product quality, such as paints, cosmetics, and pharmaceuticals.
- Considerations: Proper sample preparation is necessary. The presence of multiple particle sizes within a sample can complicate the interpretation of results.
- Capable of measuring a wide range of sizes, from nanometers to millimeters.
- Rapid measurement speeds make it suitable for high-throughput environments.
- Non-destructive analysis preserves the sample for subsequent testing.
Overall, laser diffraction offers a robust method for particle size analysis but requires an understanding of its operational parameters to optimize performance.
Dynamic Light Scattering
Dynamic light scattering (DLS) is another advanced method, often used for measuring the size of nanoparticles or colloids in a solution. In this technique, the motion of particles is analyzed as they scatter light from a laser. The speed of this motion provides insights into particle size through the Stokes-Einstein equation.
- Advantages:
- Applications: Commonly used in biotechnology and nanotechnology for analyzing drug delivery systems and other applications involving small particles.
- Considerations: The technique typically accommodates particles in the submicron range; larger particles may not yield accurate results. Additionally, sample concentrations should be carefully controlled to avoid multiple scattering effects.
- Extremely sensitive to changes in particle size, making it ideal for detecting variations in heterogeneous samples.
- Quick results, often within minutes.
Future Trends in Particle Size Analysis
The landscape of particle size analysis is continuously evolving, driven by advancements in technology and increasing demands across various fields. Understanding future trends in this area is critical, not just for researchers but also for industry professionals aiming to stay ahead of the curve. As applications become more complex and the need for precision intensifies, the role of particle size analysis will only grow more significant. This section highlights emerging technologies and digital integration that promise to reshape the methodologies associated with particle size characterisation.
Emerging Technologies in Sieve Analysis
Recent innovations are paving the way for more efficient and accurate methods in sieve analysis. One remarkable advancement is the use of automated sieving systems, which minimize human error and streamline the analysis process. With fully automated machines, the tedious task of manual sieving is transformed into a quick and precise operation. These systems often come equipped with sensors that automatically log data, allowing for real-time feedback and adjustments.
Another noteworthy development involves 3D imaging technology. This method provides a more detailed view of particle shape and distribution, going beyond what traditional sieves can offer. While conventional methods only allow for dimensional grading, 3D imaging can analyze and portray the irregularities in particle form and additional characteristics that might affect the processing and performance of materials.
Moreover, the integration of nanotechnology into particle size analysis has opened up discussions about its potential. As materials at the nano-scale become more prevalent, traditional sieving techniques may struggle to keep up. Emerging technologies tailored for the analysis of nanoparticles are crucial. They not only enhance accuracy but also provide a deeper understanding of material behavior at the micro-level.
"In the world of materials science, understanding particle characteristics at finer scales unlocks new potentials for innovative applications."
Integration with Digital Solutions
The integration of digital solutions into particle size analysis represents a significant trend worth examining. The advent of cloud computing and the Internet of Things (IoT) has led to the development of smart sieving systems. These innovative systems can communicate data and insights directly to a centralized platform, allowing for better tracking and documentation of results.
Data generated through sieve analysis can now be stored and analyzed in large databases, making predictive analytics a reality in this field. This offers real benefits such as improved quality control and compliance with regulatory standards in industries such as pharmaceuticals and food production.
The future will also likely see a rise in machine learning algorithms that analyze historical data from particle size analyses. Not only will this lead to more precise measurements, but it will also assist researchers in identifying patterns and correlating size distributions with physical properties. As a result, the data-driven approach will enhance decision-making processes, ultimately pushing innovation forward.
Such trends cannot be ignored. They illustrate the convergence of traditional methods with cutting-edge technology, a synergy that promises to enhance the quality and reliability of particle size analysis.
In sum, the future of particle size analysis using the sieve method holds great promise, driven by emerging technologies and the seamless integration of digital solutions that collectively advance our understanding and application of these techniques in various disciplines.
Closure
In reflecting on the complexities and nuances of particle size analysis via the sieve method, it's clear that this technique stands as a cornerstone in various scientific disciplines. The significance of understanding particle size cannot be overstated; it influences everything from the behavior of materials in a manufacturing context to the efficacy of pharmaceutical compounds in medical applications. As we delve into the finer points of this analysis, certain key elements emerge as paramount: accuracy in sieve calibration, the relevance of appropriate sieve types for specific materials, and the inherent limitations faced during the process.
One prominent benefit of utilizing the sieve method is its adaptability across fields such as pharmaceuticals, construction, and food science. This versatility allows researchers and professionals to tailor their approach, ensuring the results are meaningful and applicable to their specific needs. Furthermore, understanding the sieve methodology provides deeper insights into how material properties can be controlled and designed, thus allowing for innovations in product development and quality control processes.
However, while the sieve method offers numerous advantages, it is imperative to remain cognizant of its limitations. Factors such as particle shape, moisture content, and sample homogeneity can significantly impact the results, highlighting the need for careful preparation and consideration of supplementary analysis methods. The accuracy of measurements hinges not only on the equipment but also on the user's expertise.
"The journey of understanding particle sizes is not just about the numbers; it’s about leading to innovations that shape our world."
Overall, the synthesis of insights from this article underscores the necessity for continued exploration and refinement of the sieve method. As technology advances and new methods emerge, integrating these developments into traditional sieve analysis will likely enhance its efficacy and broaden its applicability.
Summary of Key Insights
- The sieve method is crucial in many scientific domains, significantly influencing material performance.
- Accurate calibration and selection of appropriate sieve types are vital for meaningful results.
- The method's versatility allows for tailored approaches across various industries.
- Awareness of limitations can help mitigate errors and improve analysis accuracy.
- Ongoing exploration in this field can lead to refined methodologies and innovations in practice.
Implications for Future Research
Future research should aim at pioneering new technologies that augment the classical sieve method. With the rapid growth in digital technologies, integrating sensors and automation into sieve analysis may enhance accuracy and efficiency. For instance, utilizing image analysis and machine learning can help in better categorization and interpretation of particle size distributions.
Moreover, understanding the interplay between particle size and material properties could unveil new avenues for industrial applications. Investigating the impact of particle shape and distribution on flow behavior in powders, for example, could inform better practices in industries like pharmaceuticals and construction.
Collaboration between disciplines, such as material science and computer science, will be essential. By pooling resources and knowledge, researchers can create innovative solutions that address current limitations while paving the way for next-generation particle size analysis techniques.