Exploring Photoelectric Light Technology: Principles and Applications
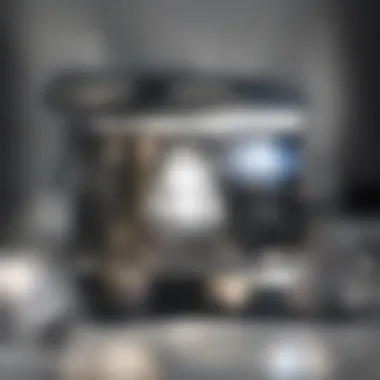
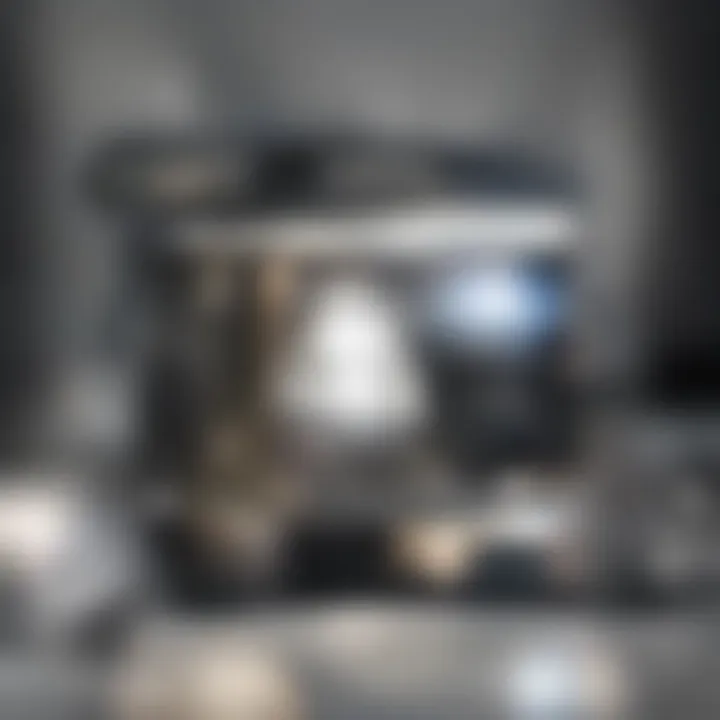
Intro
Photoelectric light technology plays a significant role in many modern devices. It uses the photoelectric effect, a phenomenon where electrons are emitted from materials when they absorb light. This technology is present in a range of applications, from solar panels to various sensors. Understanding the fundamental principles behind photoelectric effects is crucial for advancements in energy efficiency and sustainable practices.
Research Context
Background and Rationale
The significance of exploring photoelectric light technology comes from its increasing relevance in today’s society. As the world shifts focus to renewable energy sources, photoelectric systems offer promising solutions. The ability to convert light energy into electrical energy has transformative potential. Exploring its underlying physics can shed light on future innovations.
This technology serves as a backbone for numerous applications. For example, solar panels utilize the photoelectric effect to harness sunlight. This is not just about energy production but also about addressing global energy concerns and climate change.
Literature Review
Research on the photoelectric effect dates back to the early twentieth century, notably with contributions from Albert Einstein. Many academic papers discuss its principles and applications. Noteworthy studies have examined the efficiency of solar cells and the integration of this technology into various sectors.
Key publications include articles from the Journal of Applied Physics, which discuss advances in material science related to photoelectric devices. Additionally, review articles often highlight the need for efficient conversion systems, addressing the challenges faced by current technologies. One can also find insightful discussions on platforms like Wikipedia and Britannica.
Methodology
Research Design
This article employs a comprehensive literature synthesis approach. By reviewing studies, we focus on experimental findings and theoretical perspectives regarding photoelectric technology. Understanding the existing body of knowledge helps frame the discussions on current challenges and advancements.
Data Collection Methods
Data was gathered from peer-reviewed journals, articles, and online academic resources. Aggregating information from multiple sources, we analyze quantitative and qualitative data. This allows us to present a rounded view of the topic.
Closure
Prelude to Photoelectric Light
Understanding photoelectric light technology is crucial in today’s scientific and technological landscape. This field intersects various domains, from renewable energy to electronics. The advent of photoelectric technology has marked a significant shift in how we harness and utilize energy, which is particularly relevant given the current emphasis on sustainability. Information regarding photoelectric effects not only enriches academic discourse but also provides practical insights for industries reliant on light technologies.
Defining Photoelectricity
Photoelectricity refers to the phenomenon where electrons are emitted from a material as a result of incident light. This effect is fundamentally tied to the interaction between photons and electrons within materials. When light strikes a substance, it can impart energy to electrons, resulting in their liberation from the atomic structure. The efficiency of this process can greatly vary based on factors like the wavelength of light and the type of material involved.
The photoelectric effect was quantitatively explained by Albert Einstein in 1905, which played a pivotal role in the development of quantum theory. This scientific framework bridged classical physics and modern physics, revealing essential insights into atomic and subatomic behavior. Today, photoelectric principles are foundational to numerous applications, including solar panels and sensors.
Historical Context and Evolution
The history of photoelectricity is rich and encompasses significant milestones in scientific thought. It began with earlier observations of light's interaction with matter, but it was not until the late 19th century that more structured studies emerged. Heinrich Hertz first discovered the photoelectric effect in 1887. He noted that ultraviolet light could induce sparks in an electrical circuit, signaling an interaction between light and electric charge.
Einstein’s contribution in the early 20th century was revolutionary. He proposed that light is made of quantized packets of energy, known as photons. This notion fundamentally altered the way scientists understood light and matter, leading to significant advancements in technology. Over decades, photoelectric devices have evolved from rudimentary sensors to sophisticated instruments, driving improvements in fields such as telecommunications and renewable energy.
The photoelectric effect is not just a scientific curiosity; it is a vital principle behind many modern technologies, exemplifying the fusion of fundamental science with practical applications.
The Physics Behind Photoelectric Effects
The examination of photoelectric effects is essential in understanding the interaction between light and matter. This section elucidates the principles that govern these interactions, which are fundamental to many applications of photoelectric technology. Understanding the physics behind these effects not only facilitates advancements in technology but also fosters deeper insights into the nature of light itself. The interplay of photons with electrons is critical to innovations in fields like solar energy, optical sensors, and beyond.
Einstein's Contributions to Photoelectric Theory
Albert Einstein's contributions to the photoelectric effect can not be overstated. His pioneering work in the early 20th century established a theoretical framework that explained how light can eject electrons from a material. Before Einstein's insights, the wave theory of light was predominant. He proposed that light is made up of discrete packets of energy, called photons.
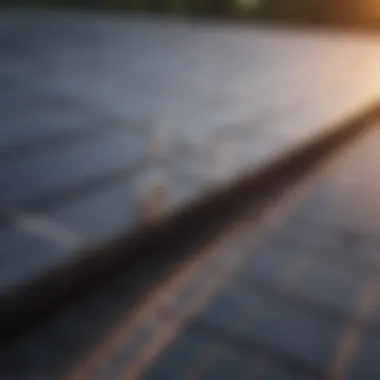
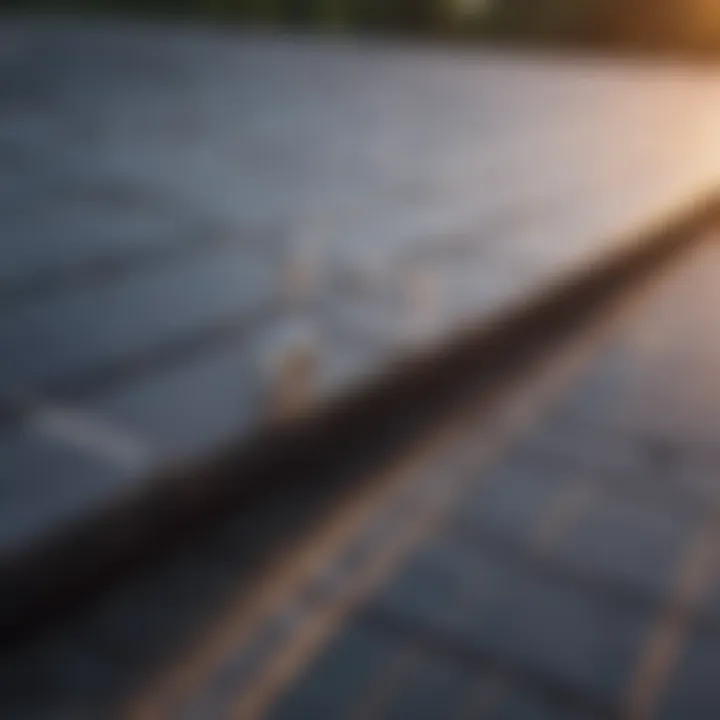
This notion led to the understanding that each photon has a specific energy, which is determined by its frequency. In simple terms, if the energy of a photon is sufficiently high, it can overcome the work function of an electron in a solid, thus liberating it from the material. This breakthrough not only provided the basis for the field of quantum mechanics but also won Einstein the Nobel Prize in 1921, solidifying his role as a key figure in modern physics.
Quantum Mechanics and Light Interaction
Quantum mechanics offers a profound understanding of how light interacts with matter. At the heart of the photoelectric effect is the principle that light exhibits both wave-like and particle-like properties. This dual nature is critical in understanding how photons can influence the behavior of electrons.
When light hits a surface, it can transfer energy to the electrons within that surface. If the energy is sufficient, the electrons will escape. Factors such as the intensity of light and the properties of the material affect this interaction. Notably, the photoelectric threshold frequency is a key concept. Light below this frequency cannot eject electrons, no matter how intense it is. This phenomenon demonstrates the quantized nature of energy interactions and redefines classical concepts of light.
Photoelectric Effect: Cause and Effect
The photoelectric effect can be described as a clear cause-and-effect relationship where a specific condition leads to a distinct outcome. In this context, when light of adequate energy strikes a photo-sensitive material, it causes the emission of electrons. This emission can be measured and analyzed, giving rise to various applications, including the design of photoelectric sensors and photovoltaic cells.
The efficiency of this effect depends largely on the specific characteristics of the material used. For example, metals such as cesium and sodium have lower work functions, making them more effective in the photoelectric process when compared to other materials. Understanding these nuances allows researchers and engineers to optimize materials used in technological applications, leading to enhanced performance and energy efficiency.
"The photoelectric effect is not just a phenomenon; it is the gateway to advancing modern technology."
This detailed grasp of the physics behind photoelectric effects not only enhances our understanding but also drives innovation in numerous applications across various fields, making it a critical area of study.
Types of Photoelectric Devices
The realm of photoelectric devices is crucial in understanding the broader principles of photoelectricity. These devices harness light energy to produce electrical signals or readings, making them integral to modern technology. Their importance extends to various applications in sectors such as renewable energy, consumer electronics, and medical diagnostics. This section focuses on three main types: Photovoltaic Cells, Photoresistors, and Photomultiplier Tubes. Each has distinct features, distributions of use, and benefits that contribute to their relevance in technology today.
Photovoltaic Cells
Photovoltaic cells play a key role in solar energy conversion. They convert sunlight directly into electricity through the photovoltaic effect. When light photons strike the cells, they knock electrons loose from atoms in the semiconductor material, which creates an electric current.
The most common type of photovoltaic cell is made from silicon. Silicon is abundant and has effective properties for capturing light. The development of thin-film technology has further advanced these cells, making them lighter and more flexible. This innovation helps in implementing solar panels in diverse environments, including curved surfaces.
Some key benefits include:
- Sustainability: Solar energy is renewable and helps reduce reliance on fossil fuels.
- Economic Viability: Over time, using photovoltaic systems can lead to savings on energy bills.
- Low Maintenance: Once installed, these systems require minimal maintenance, leading to long-term efficiency.
In recent years, research on increasing the efficiency of these cells has accelerated. More effective materials, such as perovskite solar cells, are emerging, showing potential for even greater energy conversion rates.
Photoresistors
Photoresistors, or light-dependent resistors, are another essential type of photoelectric device. They change resistance based on light intensity, making them useful in various applications. Their principle of operation lies in the conductive materials that change their resistance when illuminated.
Commonly made from cadmium sulfide, photoresistors are relatively inexpensive and simple to use. Their characteristics make them suitable for:
- Lighting Control: They can automatically adjust streetlights or indoor lighting based on ambient light levels.
- Safety Devices: Used in alarms, photoresistors can detect the absence or presence of light, triggering alerts.
- Consumer Electronics: Integrated into devices like cameras for light exposure adjustments.
While photoresistors are practical, they do come with limitations. Their response time can be slow, making them less suitable for high-speed applications. However, their affordability and versatility ensure they remain prevalent.
Photomultiplier Tubes
Photomultiplier tubes (PMTs) are sophisticated photoelectric devices that excel in detecting low levels of light. They work by taking advantage of the photoelectric effect to convert light photons into electrical signals, which they then amplify. PMTs consist of a vacuum tube and various electrodes. When light strikes the photosensitive surface, it emits electrons. These electrons are further accelerated and multiplied, creating a significant electrical output.
Key applications of photomultiplier tubes include:
- Medical Imaging: PMTs are utilized in diagnostic tools such as PET scans, enhancing signal detection.
- Nuclear Research: They measure light from scintillators, helping scientists understand radiation.
- Astronomy: PMTs detect faint light from distant celestial objects, contributing to astrophysical studies.
Despite their advantages, PMTs also have drawbacks. They are often sensitive to magnetic fields, which can affect their accuracy. Additionally, they are generally more expensive than simpler devices. However, their ability to detect extremely low light levels makes them unmatched in certain scientific applications.
The exploration of these photoelectric devices highlights the diversity and adaptability of technology. Continued research and development in these fields promise to increase their efficiency, reduce costs, and create new applications.
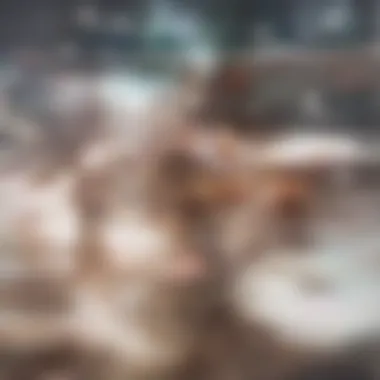
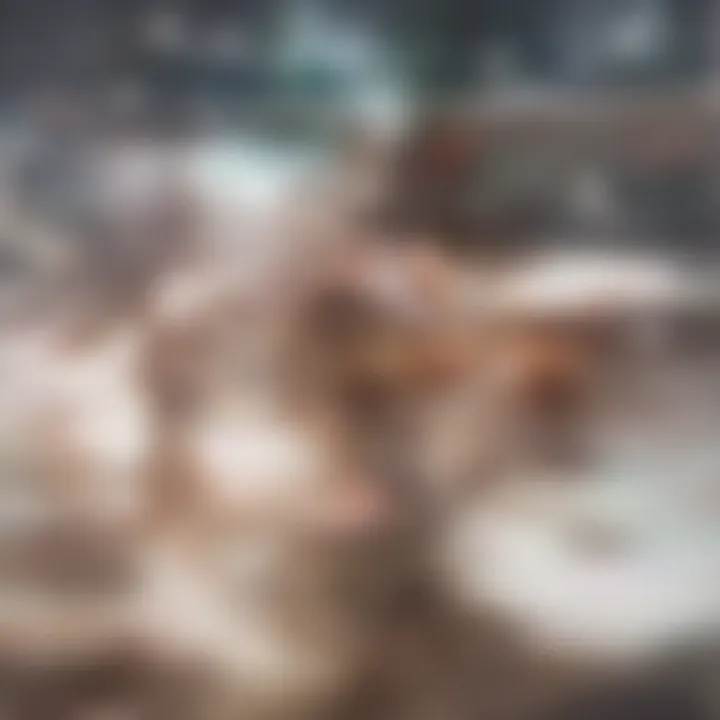
Applications in Everyday Life
The concept of photoelectric light technology has grown in significance, leading to its integration into numerous facets of daily life. Understanding its applications helps reveal its vital role in augmenting modern technology and enhancing our way of living. From household gadgets to renewable energy systems, the relevance of photoelectric devices cannot be overstated. These technologies promise benefits such as improved efficiency, sustainability, and convenience, crucial for contemporary challenges and future innovations.
Consumer Electronics
In consumer electronics, photoelectric technology is pivotal. Devices such as smartphones, cameras, and televisions utilize photoelectric sensors to enhance user experience. For instance, cameras employ photoelectric sensors to convert light into electrical signals, capturing images with remarkable clarity. Moreover, smartphones often incorporate ambient light sensors to adjust screen brightness automatically. This feature not only improves visibility but also saves battery life.
Key benefits of photoelectric applications in consumer electronics include:
- Efficiency: Enhancing user experience through responsive technology.
- Convenience: Reducing manual adjustments needed for optimum operation.
- Integration: Merging seamlessly with existing technology, leading to improved functionality.
Renewable Energy Solutions
Photoelectric technology forms the cornerstone of renewable energy solutions, particularly in solar energy systems. Photovoltaic cells, a primary component of solar panels, utilize the photoelectric effect to convert sunlight into electricity. As nations pivot towards sustainable energy sources, the relevance of these technologies escalates.
The advantages of employing photoelectric technology in renewable energy include:
- Sustainability: Using sunlight, a renewable resource, reduces reliance on fossil fuels.
- Cost-effectiveness: Over time, solar energy can significantly lower electricity bills.
- Scalability: Systems can be installed at various scales, from small residential units to large solar farms.
Medical Diagnostics
The convergence of photoelectric technology in medical diagnostics illustrates its broad applicability. Instruments such as photometers and optical sensors are essential for accurate diagnosis and monitoring. For example, pulse oximeters use a photoelectric effect to measure the oxygen saturation levels in blood non-invasively.
Some critical benefits include:
- Accuracy: High precision in measuring vital health indicators.
- Non-invasive methods: Reducing the discomfort associated with traditional diagnostic techniques.
- Real-time monitoring: Providing immediate insights into a patient’s health status.
"Photoelectric technology underpins advancements in diverse sectors, enhancing efficiency and promoting sustainability."
Research and Development Trends
Research and development in photoelectric light technology is crucial. This sector is growing, owing to the increased demand for energy-efficient solutions. Enhanced efficiency can lead to lower costs and greater adoption rates. Advances in materials and methods are making photoelectric devices more practical and accessible. As researchers delve into new technologies, the potential applications expand.
Innovations in Efficiency
Recent innovations have focused on improving the efficiency of photoelectric devices. New materials, such as perovskite solar cells, have shown remarkable promise. They can be produced at a lower cost while achieving a high conversion efficiency. Additionally, advances in nanotechnology allow for better light absorption. This results in a higher yield of energy produced from sunlight.
It is essential to consider how this efficiency impacts the environment and economy. Higher efficiency means less energy waste, which translates to more sustainable practices. There are also financial implications. Reducing production costs and improving performance can enhance market competitiveness.
Integration with Smart Technology
The synergy between photoelectric technology and smart technologies is becoming more evident. Devices can be integrated into smart grids and home automation systems, enhancing their usability. For instance, solar panels can communicate with home energy management systems. They adjust energy usage based on real-time data, increasing efficiency.
There is a rising trend toward the Internet of Things (IoT). This allows photoelectric devices to interact seamlessly with various networks and systems. As IoT frameworks advance, the capabilities of photoelectric devices will grow. They will become smarter, able to predict energy needs and optimize performance accordingly.
"The integration of photoelectric technology with smart systems represents a significant leap toward sustainable energy management."
Future Implications of Photoelectric Tech
The future of photoelectric technology holds significant implications for various sectors. This section explores two crucial aspects: sustainability and environmental impact, as well as economic considerations. Both elements are vital for understanding the trajectory of photoelectric systems in our economies and societies.
Sustainability and Environmental Impact
Sustainability is a pressing concern in today's technological landscape. Photoelectric technologies, especially photovoltaic cells, offer a pathway toward cleaner energy production. By converting sunlight directly into electricity, these systems can reduce reliance on fossil fuels.
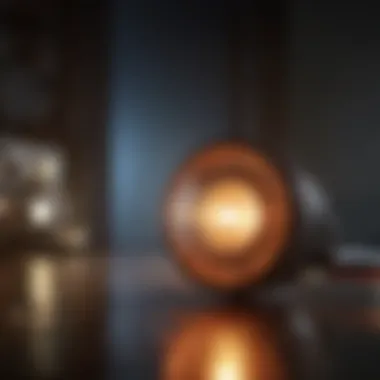
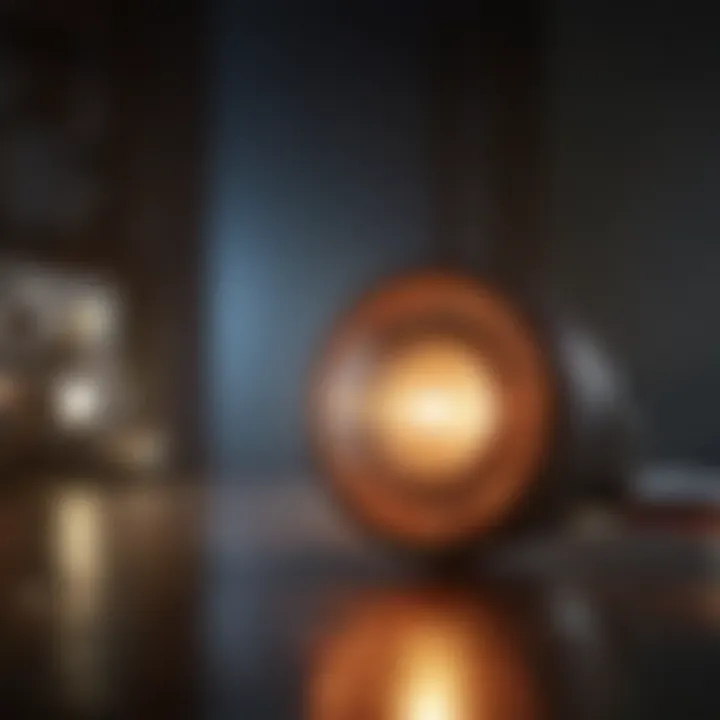
The environmental benefits are notable. When photovoltaic cells are utilized in place of conventional energy sources, there is a considerable decrease in carbon emissions. This transition contributes to global efforts in combating climate change. Furthermore, photoelectric systems are often more efficient in their use of land and resources compared to traditional energy generation methods. For instance, solar farms can be installed on previously disturbed land, minimizing habitat disruption.
However, it is important to consider the lifecycle of photoelectric devices. The manufacturing processes and end-of-life disposal must also prioritize sustainability. Recycling programs for solar panels and other devices can mitigate waste and ensure a more circular economy.
Investing in infrastructure that supports such recycling initiatives is essential for enhancing the sustainability impact of photoelectric technology.
Economic Considerations
The economic implications of photoelectric tech are multifaceted. First, the reduction in energy costs is a key benefit. As more businesses and households adopt photoelectric systems, the demand for increasingly affordable solar panels and components rises. This trend can stimulate job creation in the manufacturing and installation sectors, providing new opportunities for the workforce.
Investment in photoelectric technologies can also lead to energy independence. Regions or countries with abundant sunlight can capitalize on local solar resources, reducing vulnerability to fluctuations in energy prices and supply chain dependencies. While initial setup costs might be high, long-term savings on energy bills can offset these expenses.
However, there are challenges that can hinder widespread adoption. Market fluctuations, regulatory hurdles, and initial capital requirements can create barriers. Governments must create supportive policies that encourage investments in photoelectric technologies while ensuring fair competition in energy markets.
In summary, the future implications of photoelectric technology encompass both sustainability efforts and economic growth potential. This dual focus can shape policies and technologies that align with global objectives of reducing carbon footprints while fostering economic resilience.
Challenges and Limitations
Understanding the challenges and limitations associated with photoelectric light technology is crucial. This technology has advanced significantly, yet several factors hinder its growth and widespread adoption. These hurdles can shape the trajectory of future research and applications. Examining these challenges aids in identifying areas for improvement as well as innovations that may enhance the efficacy of photoelectric devices in various sectors.
Technical Constraints
Technical constraints pose significant barriers to the effectiveness of photoelectric light technology. One major issue is the efficiency of conversion processes. Currently, many photovoltaic cells convert only a fraction of solar energy into usable electricity. For example, silicon-based solar cells typically reach efficiencies around 15-20%. This means that a considerable amount of potential energy is wasted.
Moreover, temperature sensitivity affects the performance of photoelectric devices. Higher temperatures can lead to reduced efficiency and effectiveness, which presents challenges for deployment in hotter climates. The durability of materials used also contributes to performance limitations. Degradation caused by environmental factors can diminish the lifespan of devices, requiring costly replacements.
Additionally, integration with existing infrastructure often proves difficult. Many systems require significant modifications to accommodate new technologies. This need for adaptation can increase costs and complicate implementation, which may deter businesses and consumers from investing in photoelectric solutions.
Market Adoption Issues
Market adoption of photoelectric technology faces several obstacles. Foremost among these is the economic aspect. Many consumers and businesses are concerned about the initial costs associated with purchasing and installing photoelectric systems. Even though long-term savings are a selling point, the upfront investment may frighten away potential adopters. This is especially true for small businesses or individuals with limited budgets.
In the corporate landscape, competition also plays a role. For example, traditional energy sources, such as fossil fuels, often benefit from established infrastructure, market presence, and government subsidies. This unequal playing field makes it challenging for photoelectric technologies to gain footholds in certain markets.
Regulatory hurdles further complicate the situation. Policies and standards must evolve to accommodate and promote the use of photoelectric solutions. In many regions, lack of supportive regulations stifles potential growth.
The challenges in adopting photoelectric light technology highlight a critical juncture in the advancement of renewable energy solutions. Without addressing these limitations, progress may stall.
End
The conclusion of this article encapsulates the key themes and insights regarding photoelectric light technology. As we navigate the complexities of how photoelectric effects influence modern society, it becomes clearer that the significance of this technology cannot be overstated. Photoelectric systems represent a cornerstone in renewable energy discussions, showcasing their vast potential in a world increasingly conscious of environmental sustainability.
Summarizing Key Points
In summary, this article has examined various dimensions of photoelectric light technology, emphasizing aspects such as:
- Fundamental Principles: Understanding the physics behind photoelectricity sheds light on its functioning and applications.
- Diverse Applications: From solar panels to medical devices, photoelectric technology is integrated across numerous fields, enhancing everyday life.
- Research Trends: Innovations in efficiency and smart technology integration signal a future where photoelectric systems play a more prominent role.
- Challenges and Limitations: Identifying market adoption issues and technical constraints is crucial for future advancements in this area.
This synthesis aids researchers, students, and professionals in grasping the broader implications and relevance of photoelectric technology in contemporary society.
Call to Action for Further Research
As the realm of photoelectric technology continues to evolve, it is imperative that further research is encouraged. Consider the following avenues:
- Enhancing Efficiency: Identifying new materials or methods to improve the efficiency of photovoltaic cells could revolutionize energy consumption.
- Innovative Applications: Exploring additional uses for photoelectric devices in fields like automotive technology or environmental monitoring can yield significant insights.
- Addressing Limitations: Investigate strategies to overcome market adoption hurdles, such as developing cost-effective production methods.
Engaging in these research areas will not only expand knowledge but also promote sustainable solutions to global challenges. The exploration of photoelectric technology is a continuous journey, and collaboration among students, researchers, and professionals is vital for unlocking its full potential. >
"Investing in research and development of photoelectric technology will pave the way for sustainable energy solutions. Collaboration is the key to progress."
To conclude, photoelectric light technology holds great promise for the future, and a commitment to ongoing research can ensure its impactful role in society.