Exploring the Specific Defenses of the Immune System
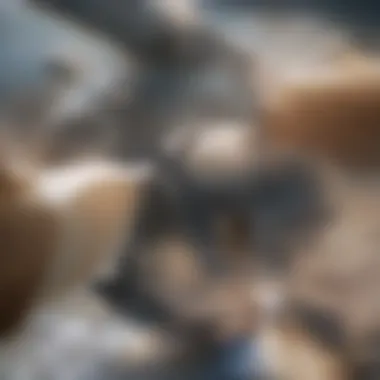
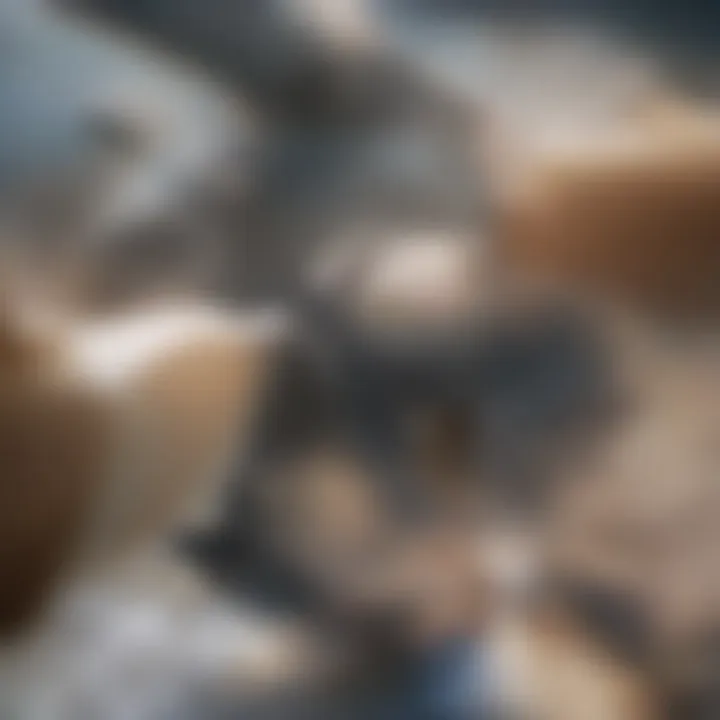
Intro
The immune system is not a monolithic entity; it operates through a complex network of specific defenses tailored to recognize and combat infectious agents. This intricate dance of cellular responses is primarily organized into adaptive immunity, a distinctive aspect of the immune response characterized by its ability to adapt and remember specific pathogens. Understanding these mechanisms can shed light on how the body protects itself from diseases and can navigate the challenges posed by new and evolving pathogens.
Research Context
Background and Rationale
The immune system has evolved over millions of years, resulting in a highly sophisticated network of cells and molecules. Specific defense mechanisms within the immune system are crucial for distinguishing between self and non-self. This discrimination is integral in protecting against infections while preventing damage to the hostโs own tissues. The rationale behind exploring these specific defenses lies in their relevance to both health and disease. Diseases such as autoimmune disorders, allergies, and cancer provide enduring implications of impaired immune responses.
Literature Review
Research has extensively detailed the components of adaptive immunity, such as T cells, B cells, and antigen-presenting cells. Studies have elucidated the processes of antigen recognition and the formation of immunological memory, which is vital for long-term immunity. A notable piece of literature is the recent review in Nature Reviews Immunology, which discusses advances in understanding how memory T cells facilitate rapid responses upon re-exposure to pathogens. Other studies highlight innovations in vaccine development that leverage these specific immune pathways to provide better protection against diseases.
Immune Cells and Their Roles
Understanding the specific cells involved is pivotal. B cells and T cells are central to adaptive immunity, with distinct yet complementary functions. B cells produce antibodies, while T cells can directly kill infected cells or assist other immune cells. Each cell type has its unique development pathway and activation process, and both are essential for a robust immune response.
- B Cells: They arise from the bone marrow and undergo a maturation process before entering circulation. Once exposed to an antigen, they differentiate into plasma cells that secrete antibodies or become memory B cells that provide long-lasting immunity.
- T Cells: These cells develop in the thymus and include a range of subtypes like helper T cells and cytotoxic T cells. Helper T cells activate other immune components, while cytotoxic T cells directly address infected cells.
Importantly, both cell types work together to enhance the specificity and efficacy of the immune response.
Antigen Recognition
Antigen recognition is the cornerstone of the specific defense mechanism. Each immune cell has receptors that identify particular antigens, which are unique markers present on pathogens. This recognition triggers the immune responses that eliminate the invader. Understanding how immune cells recognize and respond to antigens is key to comprehending diseases and developing immunotherapies. A deeper knowledge of the molecular interactions involved aids in developing better vaccines and treatments.
Immunological Memory
One remarkable feature of the immune system is its ability to remember previous infections. This immunological memory allows for a faster and more robust response upon re-exposure to the same pathogen. Memory B cells and T cells persist long after the initial infection, ready to spring into action when needed. This concept has been instrumental in the development of vaccines, which prime the immune system to recognize future threats without causing disease.
"Immunological memory is what makes vaccinations effective against various infectious diseases, enabling quicker responses against previously encountered pathogens."
Implications of Specific Defenses
Epilogue
Intro to the Immune System
The immune system serves as the body's defense mechanism against pathogens, including viruses, bacteria, and other foreign entities. Its functioning is crucial for maintaining health and preventing diseases. Understanding the immune system is essential for various fields, including medicine and public health, as it lays the groundwork for developing vaccines and treatments that enhance immune responses. With the constant evolution of pathogens, the immune system must adapt to recognize and eliminate these threats efficiently.
A well-functioning immune system depends on a complex network of cells, tissues, and organs that work in concert to provide protection. This network is divided into two primary components: innate and adaptive immunity. Recognizing the differences and interactions between these two arms of immunity is pivotal for grasping how the body protects itself.
In this article, we will delve into the specifics of adaptive immunity, showcasing its various cells and mechanisms. By exploring this intricate system, readers should gain a clearer understanding of how the immune system adjusts its responses to target specific pathogens effectively. The implications of these processes are significant, influencing health and disease management.
Overview of Immune Defenses
The immune defenses involve multiple layers of protection that actively work to identify and neutralize pathogens. These defenses are crucial not only for survival but also for maintaining overall health. Immune cells patrol the body continuously, ready to respond at a moment's notice. The primary components of immune defenses include physical barriers, innate immune responses, and adaptive immune mechanisms.
Physical barriers, such as the skin and mucous membranes, act as the first line of defense. If these barriers are breached, the innate immune system takes over and responds rapidly. Innate immunity includes responses that do not require prior exposure to a pathogen, making them immediate and consistent. These may involve phagocytic cells, such as macrophages and neutrophils, which engulf and digest pathogens.
In contrast, the adaptive immune response is more specialized. This system recognizes specific antigens and mounts a tailored response. Key players in adaptive immunity are B and T lymphocytes, which discern and remember previously encountered pathogens, ensuring a quicker response upon subsequent exposures.
Distinction Between Innate and Adaptive Immunity
The distinction between innate and adaptive immunity is fundamental to immunology. Innate immunity is characterized by its rapid response and lack of specificity. For example, when an injury occurs, innate immune cells aggregate to the site and initiate a generalized inflammatory response. The components involved, such as cytokines and chemokines, work together to recruit additional immune cells to the area.
On the other hand, adaptive immunity requires time to develop. It engages specific antigens that distinguish one pathogen from another. T lymphocytes, for example, recognize these antigens presented by Major Histocompatibility Complex molecules on the surface of other cells. This recognition prompts a targeted attack, often leading to the elimination of the pathogen and the creation of memory cells for future protection.
Ultimately, while innate immunity provides immediate defense, adaptive immunity tailors responses to particular threats, enhancing the bodyโs long-term protection. Both systems interplay effectively, ensuring a robust immune response throughout various challenges to health.
Components of Adaptive Immunity
The components of adaptive immunity are essential to the overall functionality of the immune system. This system is distinguished by its ability to specifically recognize and remember pathogens, leading to a more efficient response upon subsequent exposures. The importance of these components lies in their unique roles that contribute to long-lasting immunity and protection against various diseases.
Adaptive immunity is mediated by distinct types of white blood cells, namely B lymphocytes and T lymphocytes. Each of these cell types has specific functions that are crucial for mounting an appropriate immune response. Recognizing and understanding these components allows researchers and practitioners to appreciate how the immune system tailors its defenses to various threats, ultimately improving approaches to disease management and therapeutic interventions.
B Lymphocytes and Antibody Production
B lymphocytes, or B cells, are vital for humoral immunity, which involves the production of antibodies. When B cells encounter a specific antigen, they undergo activation and differentiation into plasma cells. These plasma cells are responsible for producing large quantities of antibodies, which are proteins that bind to antigens to neutralize or mark them for destruction by other immune cells.
A key characteristic of B cells is their ability to undergo somatic hypermutation and affinity maturation, processes that refine their antibody's binding capacity. This increases the effectiveness of the immune response with each subsequent exposure to the same pathogen. The versatility of B cells is highly beneficial in creating a diverse repertoire of antibodies, which contributes significantly to the immune system's overall adaptive capabilities.
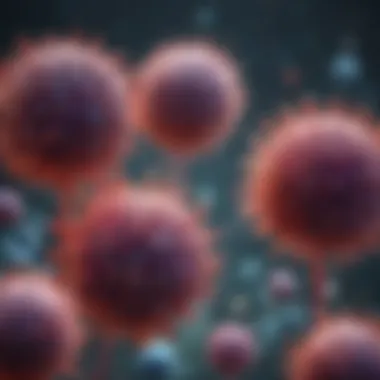
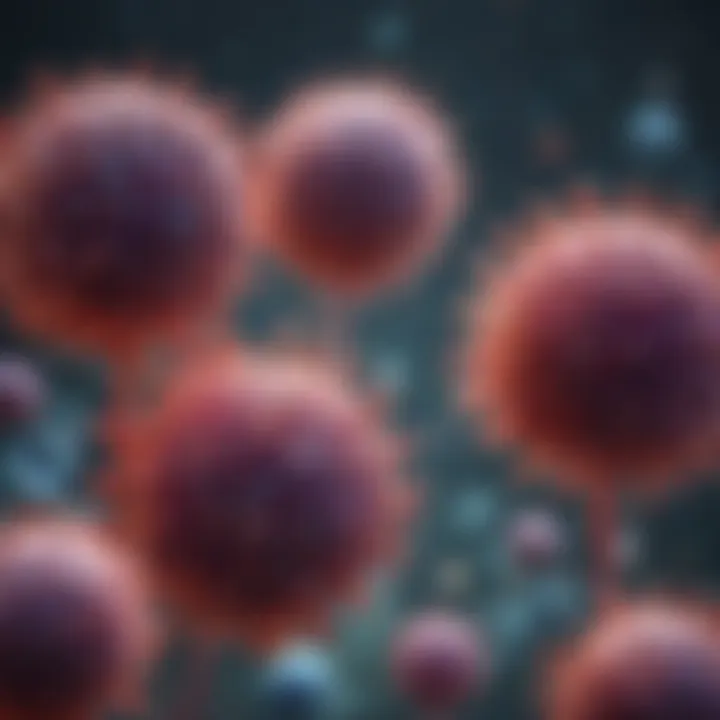
T Lymphocytes: Types and Functions
T lymphocytes play different roles essential to cellular immunity. They can be categorized into several types based on their functions:
Helper T Cells
Helper T cells, known as CD4+ T cells, are critical for orchestrating the immune response. They do not directly kill infected cells but instead help activate B cells and cytotoxic T cells. A prominent trait of helper T cells is their ability to produce cytokines, which are signaling molecules that regulate immune responses. This makes them vital for coordinating the activities of various immune components.
The unique feature of helper T cells lies in their ability to recognize antigens in the context of Major Histocompatibility Complex (MHC) class II molecules. Their activation enhances both the humoral and cellular immune responses, making them beneficial for long-term protection against pathogens. Without helper T cells, the immune system struggles to function effectively, underscoring their importance in this article.
Cytotoxic T Cells
Cytotoxic T cells, or CD8+ T cells, are crucial for directly killing infected or cancerous cells. Their main characteristic is the expression of a molecule known as CD8, which helps them recognize antigens presented by MHC class I molecules.
The ability of cytotoxic T cells to induce apoptosis in infected cells is a unique feature that proves advantageous in eliminating threats rapidly. Their specificity and efficiency make them a popular subject for discussing immune responses, especially in the context of viral infections and cancer therapies. However, overactivation can lead to tissue damage, highlighting a disadvantage that requires careful regulation of their activity.
Regulatory T Cells
Regulatory T cells, often referred to as Tregs, are fundamental in maintaining immune tolerance and preventing autoimmune reactions. A key characteristic of Tregs is their expression of CD25 and the transcription factor FoxP3, which are markers indicating their role in immune regulation.
The unique attribute of regulatory T cells is their ability to suppress the activation and proliferation of other immune cells. This feature is paramount as it helps maintain homeostasis within the immune system, preventing excessive responses that could result in damage to the host tissues. In this article, the exploration of Tregs emphasizes their significance not only in preventing autoimmune diseases but also in shaping the overall immune landscape.
Understanding the distinct roles of B and T lymphocytes in adaptive immunity provides crucial insights into how the immune system operates overall. By recognizing these components, researchers can develop targeted therapies that harness their functions effectively.
Antigen Recognition Mechanisms
Antigen recognition mechanisms are central to the functioning of the immune system and play a vital role in how the body responds to infections. The immune response requires the detection of specific proteins, known as antigens, that are present on the surface of pathogens like viruses and bacteria. These mechanisms aid not only in the identification of foreign invaders but also in the activation of appropriate immune pathways that help in eradicating them. Understanding antigen recognition is crucial for comprehending both basic immunology and advanced therapeutic strategies.
The Role of Major Histocompatibility Complex (MHC)
The Major Histocompatibility Complex (MHC) serves as an essential component in antigen recognition. MHC molecules are membrane proteins that present peptide fragments derived from proteins within a cell. There are two primary classes of MHC: Class I and Class II.
- MHC Class I molecules present antigens from intracellular pathogens. They play a role in activating cytotoxic T cells, which are essential in targeting and destroying infected cells. This is necessary particularly in viral infections when the pathogen replicates within host cells.
- MHC Class II molecules present antigens from extracellular sources. They interact with helper T cells, which then assist in the activation of various immune responses, including the proliferation of B cells and the production of antibodies.
The presentation of antigens by MHC molecules is highly regulated and can influence the specificity of the immune response. Variations in MHC genes in individuals can also impact susceptibility to infections and the effectiveness of vaccinations.
"The ability of MHC molecules to present specific antigens is a determinant for the effectiveness of the immune response and an area of immense research interest."
Clonal Selection and Expansion
Clonal selection and expansion is a critical mechanism in the adaptive immune response. When a specific antigen is recognized by a lymphocyte, such as a B or T cell, that cell undergoes clonal selection. Here is how it works:
- Recognition: A specific lymphocyte recognizes an antigen through its unique receptor, whether it be a B cell receptor or a T cell receptor. This interaction is crucial for the start of the immune response.
- Activation: Once recognized, the selected lymphocyte becomes activated. This activation may involve the provision of additional signals from helper T cells for optimal response.
- Clonal Expansion: Following activation, the lymphocyte undergoes proliferation, creating many clones of itself. These clones are all specific to the same antigen.
This expansion allows for an amplified response to a particular pathogen, ensuring that a significant number of immune cells are available to tackle the threat. After the infection is controlled, a subset of these cells remain as memory cells, playing a pivotal role in the secondary immune response. This mechanism greatly enhances the body's ability to respond more rapidly and efficiently upon re-exposure to the same pathogen.
The processes of MHC involvement and clonal selection are intricately linked within the specifics of antigen recognition. Together, they form the foundation of how the immune system adapts its response to a vast array of pathogens.
Immunological Memory
Immunological memory is a crucial aspect of the adaptive immune system, enabling an organism to respond more effectively to pathogens it has encountered previously. This capacity is vital for long-term immunity and is a primary reason vaccines are successful in preventing diseases. When the immune system first encounters an antigen, it mounts a response that includes the production of specific antibodies and the activation of immune cells. These initial responses are generally slower and less effective than those generated after the immune system has formed a memory of the antigen.
The significance of immunological memory lies in its ability to improve the quality and speed of immune responses. Memory B cells and T cells, formed during the primary immune response, remain in the body long after the initial infection has cleared. When the same pathogen invades again, these memory cells can rapidly recognize the antigen and mount a robust, swift response. This phenomenon is often the reason individuals may never contract the same illness twice or why diseases like chickenpox typically do not affect someone more than once.
"A strong immunological memory can lead to lifelong protection against certain diseases."
Additionally, this memory is not limited to individual pathogens. It can also provide cross-protection against closely related strains of a pathogen, which is significant in the context of viral infections such as influenza.
While the benefits of immunological memory are evident, several considerations should be acknowledged. The duration and effectiveness of memory responses can vary widely depending on factors like the pathogen type, age, and even genetic predispositions. Furthermore, certain pathogens possess the ability to evade memory responses through mutation, thus posing challenges to the immune system.
Primary vs Secondary Immune Responses
The immune response depends on whether it is the first encounter with a pathogen or a subsequent one. The primary immune response occurs when an antigen first enters the body. During this phase, naive B and T lymphocytes are activated. Initially, the level of antibodies is low, as the immune system needs time to identify and react to the new pathogen. This lag phase explains why individuals often experience symptoms during a first infection.
In contrast, the secondary immune response is characterized by a much faster and more robust reaction. Memory lymphocytes circulate in the body, prepared to recognize previously encountered antigens. As a result, the production of antibodies peaks rapidly, often reaching higher levels than during the primary response. This difference illustrates how immunological memory provides enhanced protection against re-infection.
Formation of Memory Cells
The formation of memory cells is a crucial mechanism by which the immune system retains information about pathogens. After the initial immune response, the survival of specific B and T cells becomes essential in creating memory cells. These cells persist in the body for years or even decades, allowing for rapid reactivation upon subsequent encounters with the same antigen.
This process begins when an antigen stimulates B and T cells, leading to their proliferation and differentiation. Some of these activated cells become effector cells, which actively combat the infection, while others undergo a transformation into memory cells. Memory B cells are specifically designed to produce antibodies with higher affinity for the antigen, thus enhancing their effectiveness. Memory T cells have various roles, such as helping coordinate immune responses and directly killing infected cells.
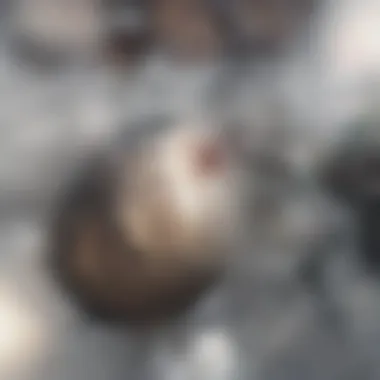
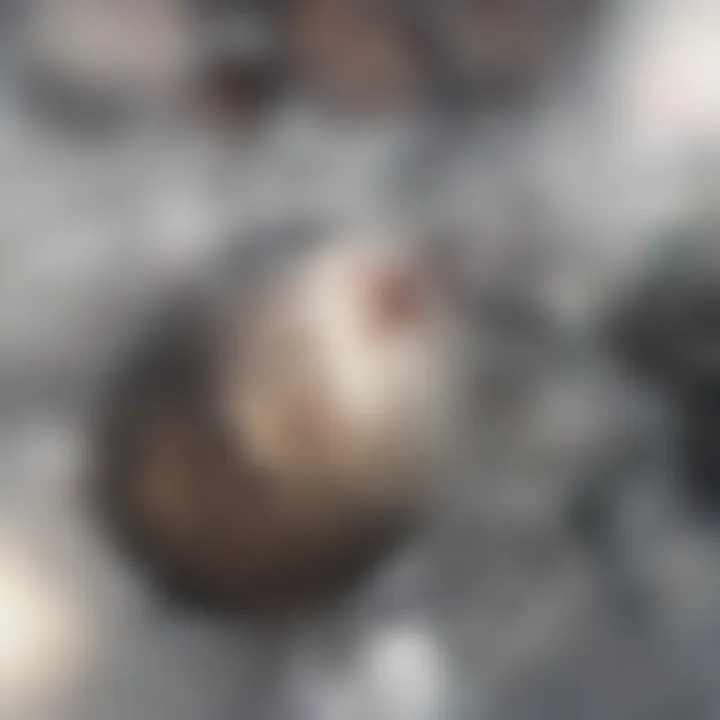
Establishing a strong reservoir of memory cells depends on several factors, including the nature of the antigen, duration of exposure, and the quality of the initial immune response. Vaccines rely heavily on this principle, aiming to trigger memory formation without causing disease, thereby equipping the immune system with the ability to respond swiftly to future infections.
Cytokines and Immune Signaling
Cytokines play a fundamental role in the immune system's functioning, acting as signals that mediate communication between immune cells. They are proteins secreted by various cells and have a crucial role in immune signaling, helping coordinate the body's response to pathogens and other immune challenges. Understanding cytokines is essential, as they not only facilitate communication within the immune system but also influence the behavior of immune cells, directing them towards specific tasks needed for combating infections.
Types of Cytokines
Cytokines are classified into several types, each serving distinct functions in the immune response. Some key types include:
- Interleukins (ILs): These are produced mainly by leukocytes and act on other leukocytes. They play diverse roles in immune regulation and cell growth.
- Tumor Necrosis Factors (TNF): This family of cytokines is involved in inflammation and apoptosis. TNF-alpha is a well-known member, primarily produced by macrophages.
- Interferons (IFNs): Interferons are critical in the defense against viral infections. They enhance the immune response by activating immune cells like natural killer (NK) cells.
- Chemokines: These are a subset of cytokines with the primary function of directing the movement of immune cells toward infection sites or sites of injury.
Each type of cytokine possesses unique characteristics and varies in function, which collectively supports the complex orchestration of immune responses. The balance among different cytokines is crucial; an imbalance can lead to immune disorders or excessive inflammation.
Regulatory Functions of Cytokines
Cytokines are not only messengers but also regulators of the immune system. Their regulatory functions include:
- Promoting Cell Differentiation: Cytokines guide the differentiation of progenitor cells into various immune cell types, which is essential for developing a robust immune response.
- Influencing Immune Cell Activation: They modulate the activation state of immune cells, ensuring that the response is adequate to the threat. For example, helper T cells release cytokines that activate B cells to produce antibodies.
- Regulating Inflammation: Many cytokines are involved in promoting or inhibiting inflammatory processes. For instance, IL-10 functions to downregulate inflammatory responses and promote tissue repair.
Important Note: The precise regulation of cytokine expression is crucial to maintaining homeostasis and ensuring effective immune responses without causing unintended tissue damage.
In summary, cytokines and immune signaling are vital components of the immune system, providing the necessary communication between different immune cells. The intricate network of cytokine interactions largely influences the outcome of immune responses, highlighting their importance in both health and disease.
The Role of Dendritic Cells
Dendritic cells are crucial players in the immune system. They function as antigen-presenting cells (APCs), bridging the innate and adaptive immunity. By capturing and processing antigens, they enable T lymphocytes to recognize pathogens effectively. This central role makes them vital for initiating immune responses. Without dendritic cells, the adaptive immunity would lack coordination and efficiency.
One of the primary functions of dendritic cells is to act as sentinels. They patrol tissues and seek out pathogens. When they encounter antigens, they ingest and process them into peptide fragments. These fragments are then displayed on their surface using major histocompatibility complex (MHC) molecules. This presentation is key because it allows T cells to recognize specific antigens. Consequently, dendritic cells also provide essential signals that help activate naive T cells.
Dendritic cells have a unique capability. They can move to lymph nodes after activating agains pathogens. In the lymph nodes, they present antigens to T cells more efficiently. Their ability to migrate is a significant advantage to the immune system. It ensures that adaptive responses can be fine-tuned to specific pathogens invading the body.
Moreover, dendritic cells are not uniform in their function. Different subsets play distinct roles. For example, some types of dendritic cells promote T or T responses, which are essential for different types of pathogen attacks. This diversity allows for more tailored immune responses, enhancing the effectiveness of defense mechanisms against various infections.
Additionally, dendritic cells can also influence the balance between tolerance and immune activation. By presenting self-antigens, they play a role in preventing autoimmune reactions. This function indicates their importance not only in immunity but also in maintaining self-tolerance, highlighting their multifaceted role in health and disease.
In summary, dendritic cells are indispensable in shaping the adaptive immune response. Their ability to process and present antigens is essential for T cell activation and subsequent immune defense.
Antigen Presentation
The process of antigen presentation by dendritic cells is vital for the activation of T cells. When dendritic cells capture antigens, they process them into peptide fragments. These fragments bind to MHC molecules on the dendritic cell surface. There are two main classes of these MHC molecules: MHC class I and MHC class II.
- MHC Class I molecules are found on all nucleated cells. They present endogenous antigens, which are typically derived from proteins synthesized within the cell. This pathway is crucial for presenting viral antigens to CD8+ cytotoxic T cells.
- MHC Class II molecules are restricted to professional antigen-presenting cells like dendritic cells, macrophages, and B cells. These molecules display exogenous antigens that have been engulfed and processed, leading to CD4+ helper T cell activation.
This distinction is significant because it determines how T cells recognize and respond to pathogens. The engagement of T cell receptors (TCRs) with the antigen-MHC complex is the first step toward activating the adaptive immune response. A successful interaction requires co-stimulatory signals provided by dendritic cells, ensuring that T cells can respond appropriately.
Activation of T Cells
Once dendritic cells present antigens, T cells can undergo activation. However, merely recognizing an antigen is insufficient for full activation. Two key signals are necessary for T cell activation:
- Signal 1 comes from the specific recognition of the antigen-MHC complex by the T cell receptor.
- Signal 2 is delivered through co-stimulatory molecules on the surface of dendritic cells. This second signal helps prevent inappropriate T cell activation, which could lead to autoimmunity.
When these signals are combined, T cells proliferate and differentiate into various subsets, including helper T cells and cytotoxic T cells. Helper T cells assist in orchestrating the immune response, while cytotoxic T cells are essential for killing infected or cancerous cells. Dendritic cells play a pivotal role in this process, ensuring that the immune system is trained to recognize and effectively respond to formidable threats.
Pathogen Evasion Strategies
Understanding how pathogens evade the immune system is crucial for grasping the dynamics of infections and the specific defensive measures of our immune system. Pathogen evasion strategies complicate the immune response, allowing various microorganisms to persist within the host. By learning about these strategies, we can appreciate the ongoing battle between immune defenses and pathogenic mechanisms.
Mechanisms Employed by Viruses
Viruses exhibit several clever strategies to escape detection and destruction by the immune system. One prominent method is antigenic variation. This means that a virus can change its surface proteins to avoid recognition by pre-existing antibodies. Influenza viruses are a classic example, where small mutations occur in their protein structures, leading to seasonal outbreaks.
Another common tactic is the direct inhibition of immune mechanisms. Some viruses produce proteins that can block the action of interferons, which are crucial for antiviral responses. This allows viruses like the hepatitis C virus to replicate efficiently within the host.
- Latency is another survival strategy. Certain viruses can enter a dormant state within cells, such as herpes viruses. During latency, they do not produce detectable proteins, allowing them to evade the immune system.
- Viral decoys are also used. Some viruses release soluble versions of their surface proteins into the bloodstream, acting as decoys that bind to neutralizing antibodies, thereby preventing these antibodies from targeting the actual virus.
These mechanisms underline the importance of continuous research in viral immunology to develop effective treatments and vaccines. The ongoing evolution of viruses demands a vigilant and adaptable immune response.
Bacterial Evasion Tactics
Bacteria have their own set of evasion strategies. Unlike viruses, which depend on host cells for replication, bacteria can thrive independently. One major tactic is the formation of a biofilm, a protective layer made of sugar-like substances. Biofilms act as shields for bacteria, making it more difficult for immune cells and antibiotics to penetrate and eliminate the infection. An example is the biofilm produced by Pseudomonas aeruginosa, often seen in chronic lung infections in cystic fibrosis patients.
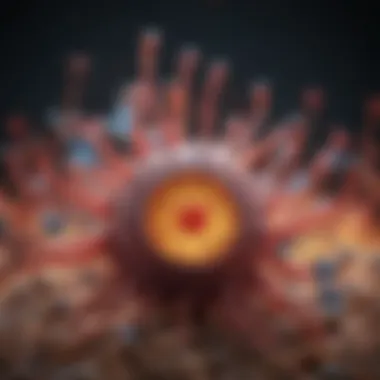
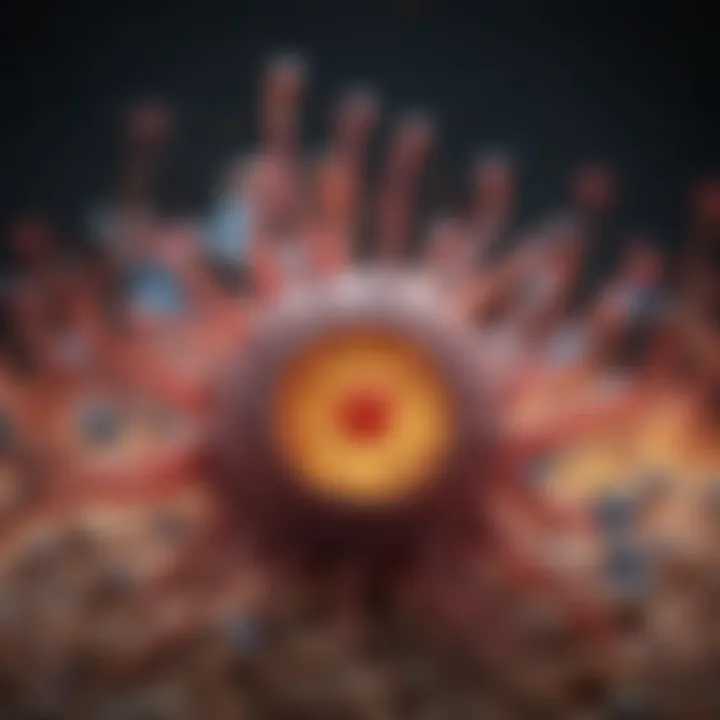
Bacteria can also employ capsules, which are external structures that protect them from phagocytosis by immune cells. The capsule acts as a physical barrier, making it hard for immune cells to engulf and destroy the bacteria. Streptococcus pneumoniae is well-known for this evasion strategy, as its capsule inhibits immune recognition.
Additionally, certain bacteria can produce proteins that disrupt immune signaling pathways. For instance, Salmonella enterica can interfere with the detection of its presence by the immune system, allowing it to survive and replicate in host cells.
- Antigenic variation is not unique to viruses. Many bacteria, such as Neisseria gonorrhoeae, can alter their surface antigens to escape the adaptive immune response.
- Lastly, some bacteria can directly modulate the host immune response, manipulating the signaling pathways to suppress activation of key immune cells. This adaptability complicates treatment strategies in bacterial infections.
Chronic infections often arise due to these bacterial evasion tactics, posing significant challenges for healthcare providers.
In summary, understanding pathogen evasion strategies allows for better prevention and treatment of infectious diseases. Continuous research is critical to keep pace with the evolving capabilities of pathogens.
Immune System Disorders
Autoimmune Diseases
Autoimmune diseases occur when the immune system mistakenly attacks the body's own healthy cells. This phenomenon highlights a significant flaw in the immune defense mechanism, as it struggles to differentiate between foreign invaders and self. There are over eighty known autoimmune diseases, including lupus, rheumatoid arthritis, and type 1 diabetes. Each of these conditions presents unique challenges, impacting millions of individuals worldwide.
Key points about autoimmune diseases include:
- Mechanism of Action: In autoimmune diseases, the immune response becomes hyperactive, leading to inflammation and tissue damage. T cells and antibodies can target critical organs, disrupting their functions.
- Impact on Quality of Life: Patients often experience chronic pain, fatigue, and other debilitating symptoms. Effective management is crucial to improve their daily living.
- Current Research Trends: Scientists are exploring therapeutic avenues, such as biologics and personalized medicine. Targeted treatments aim to modulate immune activity, providing relief for patients.
Understanding the intricacies of autoimmune diseases is essential for developing better treatment strategies. By fostering a deeper comprehension of these conditions, researchers hope to uncover new pathways that can mitigate their effects.
Immunodeficiency Disorders
Immunodeficiency disorders indicate a weakened immune system, making individuals more susceptible to infections. These disorders can be congenital or acquired. Congenital immunodeficiencies are present at birth due to genetic defects, while acquired types can develop due to factors such as malnutrition, aging, or infection, such as HIV.
Components of immunodeficiency disorders include:
- Types of Disorders: Conditions such as Severe Combined Immunodeficiency (SCID) or Common Variable Immunodeficiency (CVID) highlight the diverse landscape of immunodeficiency.
- Symptoms and Signs: Recurrent infections, poor wound healing, and unusual autoimmune involvement are common indicators of a weakened immune response.
- Therapeutic Alternatives: Treatments can involve immunoglobulin therapy, antibiotics, and even bone marrow transplants in severe cases. Ongoing research aims to enhance these strategies.
It is essential to identify immunodeficiency disorders early to safeguard patients from severe health complications.
Vaccination and Immunization
Vaccination and immunization represent critical elements of modern medicine. These processes have reshaped the landscape of public health by effectively combatting infectious diseases. Vaccination involves introducing a vaccine, which is a biological preparation that provides immunity to a particular infectious disease. Immunization is the broader process through which individuals develop immunity, either through vaccination or previous infection. The intricate relationship between these two components emphasizes their importance in safeguarding health.
Vaccination has numerous benefits. A primary benefit is the prevention of disease outbreaks. By vaccinating a large segment of the population, herd immunity can be established. This lowers the overall amount of the virus available to spread, protecting those who cannot be vaccinated, such as infants or individuals with certain health conditions. Effective vaccines can lead to the eradication of diseases, as seen with smallpox. Additionally, vaccinations reduce the economic burden associated with healthcare costs and lost productivity due to illness.
However, considerations regarding vaccination can be complex. Vaccine hesitancy has emerged as a significant public health challenge. Misinformation surrounding vaccination risks can lead to reduced uptake rates, raising concerns about the resurgence of preventable diseases. Public health campaigns must effectively communicate the science behind vaccines, emphasizing their safety and efficacy. Providing reliable information can help address fears and misconceptions in communities.
Mechanisms of Vaccines
Vaccines work through several mechanisms to provide immunity. The most common forms of vaccines include inactivated or killed pathogens, live-attenuated pathogens, subunit proteins, and messenger RNA (mRNA). Each type of vaccine stimulates the immune system differently, leading to the formation of memory cells.
- Inactivated or Killed Pathogens: These vaccines use pathogens that have been killed or inactivated. They cannot cause disease but can still stimulate an immune response, producing antibodies against that specific pathogen.
- Live-Attenuated Pathogens: These involve live microbes that have been weakened so they do not cause disease in healthy individuals. These vaccines often provoke a strong immune response due to the active infection's mimicry.
- Subunit Vaccines: These consist of purified components of the pathogen, such as proteins. They focus the immune response on those critical parts, reducing the potential for side effects compared to whole pathogen vaccines.
- Messenger RNA (mRNA) Vaccines: mRNA vaccines use pieces of the virus's genetic material to instruct cells to produce a harmless protein that is part of the virus. This triggers an immune response without involving the live pathogen.
"Vaccines are a proven method to protect individuals and communities from infectious diseases. Their successful implementation requires understanding their mechanisms and addressing concerns effectively."
Impact on Public Health
The effects of vaccination on public health are profound. Vaccination has led to a significant decline in morbidity and mortality rates related to infectious diseases. This decline can be observed in diseases such as polio, measles, and pertussis. Through widespread immunization efforts, public health systems have a greater capacity to protect vulnerable populations and control disease outbreaks.
Moreover, vaccinations have economic implications. By reducing the incidence of disease, healthcare expenses decrease, benefiting the economy at large. A healthy population contributes to productivity, positively impacting societal progress.
The global approach to vaccination has seen the establishment of initiatives like the Global Vaccine Alliance, which aims to provide equitable access to vaccines, regardless of socioeconomic status. This ensures that even the most vulnerable populations receive the protection they require.
Recent Advances in Immunology Research
Recent advances in immunology research are not only important but also transformative in understanding how the immune system functions. This field of study is rapidly evolving, revealing new therapeutic targets and strategies to enhance immune responses against various diseases. Recognizing these advances can lead to significant breakthroughs in vaccine development, treatments for autoimmune disorders, and even cancer immunotherapy. By bridging the gap between fundamental research and clinical application, immunology stands at the forefront of medical innovation.
Novel Therapeutics Targeting Immunity
The emergence of novel therapeutics focusing on immunity marks a pivotal moment in medical science. Recent innovations, such as monoclonal antibodies and checkpoint inhibitors, have revolutionized approaches to treating diseases like cancer. Monoclonal antibodies are designed to specifically target antigens present on cancer cells, facilitating immune recognition and destruction. Meanwhile, checkpoint inhibitors enhance the immune system's ability to target tumors by blocking proteins that inhibit immune responses.
Additionally, CAR-T cell therapy represents a groundbreaking approach where a patientโs T cells are genetically engineered to attack cancer cells more effectively. This personalized treatment not only advances the concept of targeted therapy but also underscores the potential of tailoring immune responses to individual patients.
"The ability to biologically engineer immune cells offers new hope for patients with previously untreatable conditions."
The synergy between immunology and biotechnology continues to unveil therapeutic strategies aimed at boosting the immune response. These developments could eventually lead to more efficacious treatments with fewer side effects compared to traditional therapies.
Role of Microbiome in Immune Function
The microbiome has emerged as a critical player in regulating immune responses. Recent research highlights that the diverse community of microorganisms residing in our bodies significantly influences immune system development and function. The interactions between gut bacteria and immune cells contribute to the establishment of a robust immune system. For instance, certain beneficial bacteria may promote the production of anti-inflammatory cytokines, enhancing the body's defense mechanisms.
Furthermore, the microbiome's composition can impact susceptibility to diseases. An imbalance in microbial communities, known as dysbiosis, has been linked to various conditions, including allergies and autoimmune diseases.
- Understanding the microbiome opens avenues for novel therapies such as probiotics, which could modulate immune responses.
- Research into microbiome-based interventions offers insights into preventing immunological disorders and improving overall health.