Understanding Geiger Counter Readings Effectively
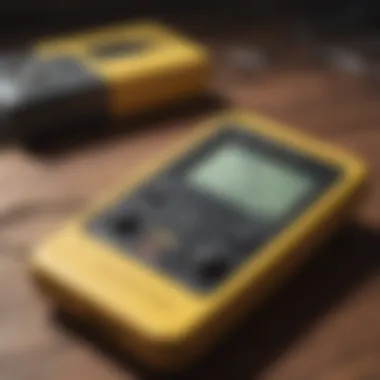
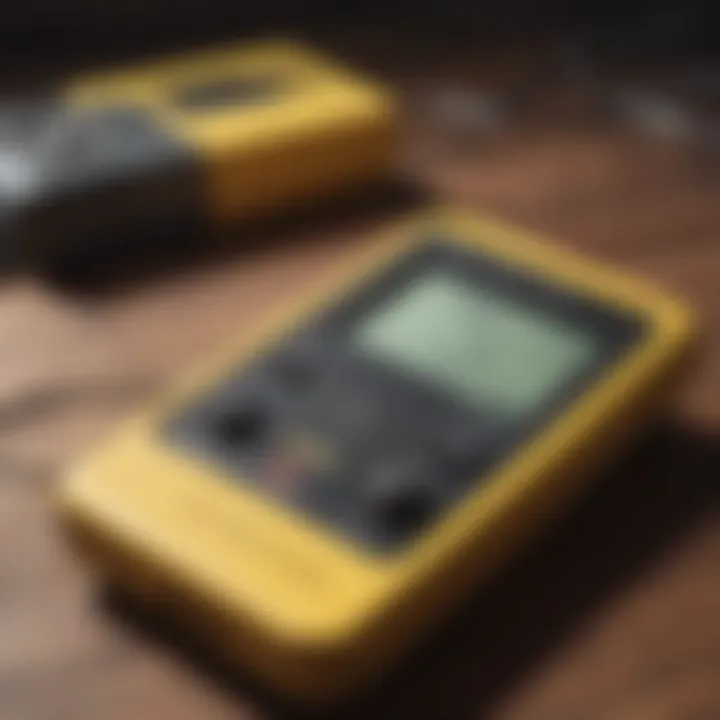
Intro
Understanding how to read and interpret the readings from a Geiger counter is essential for anyone involved in fields ranging from nuclear safety to environmental monitoring. These devices are invaluable tools used to measure ionizing radiation, making it crucial to grasp their functionality and the implications of the readings provided. As we step through the principles of Geiger counters, the kinds of radiation they detect, and the nuances in interpreting their outputs, we aim to empower users of all experience levels.
Radiation measurement is not merely a technical endeavor; it involves careful attention to detail and a thorough understanding of the underlying science. The stakes can be high, whether in a research lab or during an emergency situation. As such, our exploration here will be rooted in both scientific accuracy and practical application, ensuring that every reader, from novices to seasoned professionals, walks away with a richer understanding of these critical devices.
Research Context
Background and Rationale
The Geiger counter has been a staple since its invention in the early 20th century, emerging out of an urgent need to measure and understand ionizing radiation. With the growing use of radioactive materials in various sectors, including medicine, energy, and industry, the importance of reliably interpreting these readings has become apparent. Events like the Chernobyl disaster of 1986 or the Fukushima accident in 2011 drew sharp attention to the necessity for precise radiation monitoring. This has fueled research and development in making these instruments more accessible and accurate. Furthermore, an increase in public concern about radiation exposure has emphasized the need for educational resources aimed at helping individuals decipher Geiger counter readings in their day-to-day lives.
Literature Review
Numerous studies and publications have addressed the mechanics of Geiger counters and the nature of radiation. Papers have dissected the types of radiation—alpha, beta, and gamma—and their detection processes using Geiger-Müller tubes. Texts like "Radiation: A History of the Past and the Future" highlight the evolution of radiation detection technology. Resources available through platforms such as Wikipedia and Britannica provide foundational knowledge, while specialized journals delve into technical advancements in calibration and safety protocols.
The existing literature often skims over the practical aspects of interpreting readings, a gap this article aims to fill. By focusing on how readings are displayed, what factors might alter these readings, and best practices for calibration, we intend to offer a hands-on guide that bridges the theoretical with the practical, making this information accessible to a broader audience.
Methodology
Research Design
To effectively convey the operational intricacies of Geiger counters and the interpretation of measurements, a qualitative approach will be utilized. This approach involves synthesis of existing literature, expert interviews, and case study analysis. Each facet will contribute to a comprehensive understanding of the subject matter, with insights drawn from practical experiences in both laboratory settings and fieldwork.
Data Collection Methods
- Interviews with experts in radiation safety and monitoring
- Review of academic articles and industry reports
- Analysis of user experiences shared on platforms like Reddit and other community forums
- Examination of calibration procedures as outlined by manufacturers and regulatory bodies
This structured methodology lays the groundwork for a detailed exploration of interpreting Geiger counter readings. As we progress through the subsequent sections, it’s our goal to ensure that each reader gains the confidence needed to handle these instruments effectively and safely.
Understanding Geiger Counters
In any conversation about radiation detection, it’s imperative to graps the role of Geiger counters. These devices aren't just technical gadgets; they are essential tools that can help ensure safety in various professions and environments where ionizing radiation exists. Whether one is a scientist, a health professional, or just a curious mind, knowing how a Geiger counter operates offers a significant advantage in interpreting the potential hazards of radiation exposure.
Understanding Geiger counters involves familiarizing oneself with their definition, functionality, and historical background. It paves the way for clearer insights when reading data, crucial for decision-making in health, environmental assessments, and even nuclear energy settings. With this base of knowledge, users can critically analyze readings and respond effectively should any anomalies arise.
Definition and Purpose
At its core, a Geiger counter is a device designed to measure ionizing radiation, which includes alpha and beta particles, as well as gamma rays. Imagine it as a watchful sentry, quietly monitoring the environment for radiation levels that might otherwise go unnoticed. The main purpose of this device is not just detection, but also ensuring that individuals can operate safely in environments where radiation might be present.
A Geiger counter converts the ionization of gas within a Geiger-Müller tube into audible clicks or visual readings on a display. Each click signifies a detection event, effectively serving as an alert system for increasing radiation levels, allowing users to gauge their exposure time and act accordingly.
Historical Context
The journey of radiation detection devices began long before the age of fancy gadgets. It all started in the late 19th century, when pioneers like Marie Curie and Henri Becquerel illuminated the scientific community with their discoveries related to radioactivity. The growing understanding of radiation’s effects led to the development of many detection methods over the years, culminating in 1928 when Hans Geiger, in collaboration with Walther Müller, invented the Geiger-Müller counter.
This instrument evolved significantly, along with our understanding of the health risks posed by radiation. Initially limited to laboratory settings, it gradually made its way into industries like healthcare, nuclear energy, and environmental monitoring. Today, whether in a classroom or a hazardous site, Geiger counters are indispensable, having stood the test of time and technological advancements.
Operational Mechanics of a Geiger Counter
Understanding the operational mechanics of a Geiger counter is crucial for effectively interpreting its readings. Knowledge of how the device functions extends beyond mere usage; it allows users to appreciate the nuances of the data presented. This understanding can contribute significantly to accurate readings, especially in critical situations such as environmental monitoring or safety assessments in nuclear facilities.
Components and Functionality
Geiger-Müller Tube
The heart of a Geiger counter lies in the Geiger-Müller tube. This component is the primary area where ionization occurs, leading to the detection of radiation. When radiation interacts with the gas inside the tube, it ionizes the gas, causing an avalanche effect that produces a measurable pulse of current. The Geiger-Müller tube is popular due to its durability and effectiveness. Its key characteristic is its simplicity—responding to a range of radiation types without the need for elaborate setups.
However, a downside to consider is its limited energy sensitivity; it may not distinguish between high-energy and low-energy radiation effectively. While suitable for general radiation detection, it could lead to overgeneralizations if users aren't cautious.
Voltage Supply
The voltage supply is another vital component that enables the Geiger counter to function properly. It powers the Geiger-Müller tube and establishes the necessary conditions for ionization to occur. A notable benefit lies in the tube's ability to operate at different voltages depending on its design, which allows for flexibility in application.
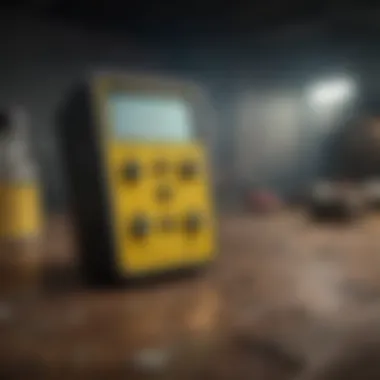
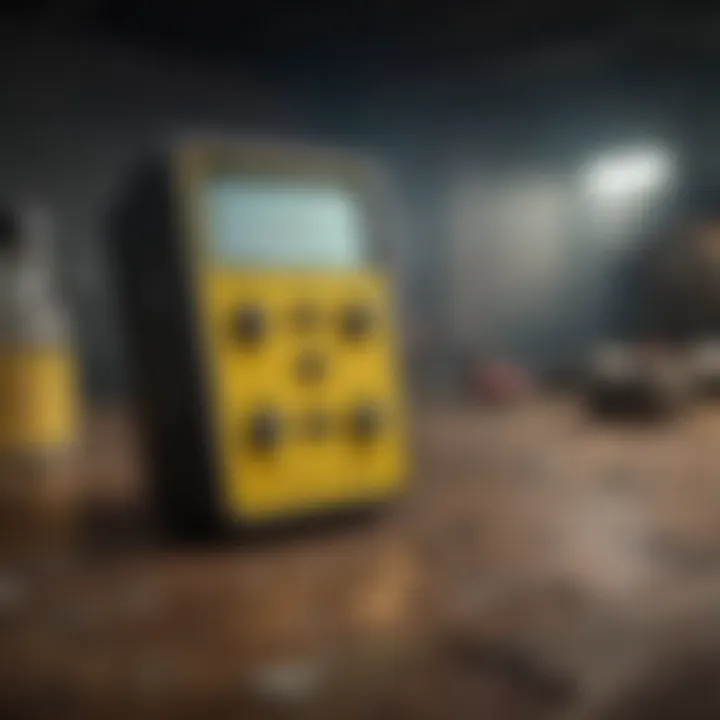
Yet, too high a voltage can cause saturation, resulting in inaccurate readings. This aspect makes the voltage supply's specification and calibration undeniably crucial in ensuring that the device operates optimally.
Counting Circuit
A counting circuit gathers the electrical pulses generated by the Geiger-Müller tube and processes them to reflect radiation levels. This circuit's main function is to compute the rate of detected ionization events within a specified time. The uniqueness of the counting circuit lies in its ability to filter and categorize counts, often providing outputs like counts per minute or a visual readout on a digital screen.
However, interpreting these counts requires an understanding of factors such as time intervals and the sensitivity of the circuit. If the counting circuit is improperly calibrated, even a correctly functioning Geiger-Müller tube can yield misleading results.
Detection of Radiation Types
Recognizing the types of radiation detected is essential for accurately interpreting readings from a Geiger counter. Each radiation type presents different challenges and implications for safety and understanding.
Alpha Particles
Alpha particles are a type of radiation that consists of two protons and two neutrons, making them relatively heavy and positively charged. They cannot penetrate the skin, which means they are less of a threat externally but can cause significant damage if ingested or inhaled. The ability of a Geiger counter to detect alpha particles offers accounts of potential contamination, which is crucial in both environmental and healthcare contexts.
However, the unique feature of alpha particles is that they require a specific window in the Geiger-Müller tube to detect them effectively. That can sometimes limit their measurement range in portable devices.
Beta Particles
Beta particles are much lighter and can penetrate human skin, making them a more serious concern in terms of radiation exposure. They are essentially electrons or positrons ejected from atomic nuclei during radioactive decay. The importance of detecting beta particles cannot be overstated; they can expose individuals and environments to risk without visible signs.
These particles also require a different tube design for optimal detection, and this adaptability is one of the advantages of modern Geiger counters. However, this may reduce the device's lifespan or create issues if frequent calibration isn't performed.
Gamma Rays
Gamma rays are highly penetrating electromagnetic radiation, characterized by their ability to traverse most matter readily. They pose unique challenges, especially in terms of shielding and detection. The Geiger counter’s capability to measure gamma rays is vital, particularly in industries dealing with nuclear materials or in emergency response situations.
While gamma rays' penetrative ability is a benefit for detection in various scenarios, it also entails challenges—specifically regarding calibration and the choice of shielding materials. Because gamma radiation can come from different isotopes, the Geiger counter still has to be tailored carefully to specific needs for accurate readings.
The varying nature of radiation types necessitates a keen awareness of their characteristics to ensure safety and understanding.
Reading the Geiger Counter
Understanding how to accurately read a Geiger counter is paramount for anyone engaged in the field of radiation measurement and safety. The readings from this device can inform critical decisions in environments ranging from laboratories to nuclear power plants. Misinterpretation, on the other hand, can lead to devastating consequences, not just for individual safety but for community health as well. This summons the need to delve into the specifics of interpreting each reading – understanding the count rates, measurement units, and the contextual factors influencing these numbers.
Interpreting Count Rate
The count rate is essentially the heart of what a Geiger counter reports. It's the raw count of ionizing events detected over a specified time period. This simple number can paint a myriad of pictures depending on the context. For example, a count rate of 30 counts per minute might be completely innocuous in a clean environment but signify something far more dangerous in a contaminated one.
More importantly, it’s vital to recognize that the readings can fluctuate throughout the day. An increase could reflect natural background radiation or signal a transient event like a nearby construction project disturbing radioactive materials. Thus, capturing an accurate count requires not just an understanding of the numbers but also a contextual lens through which the data is evaluated.
Understanding Measurement Units
In interpreting Geiger counter readings, familiarity with measurement units is non-negotiable. Here we will explore three primary units you will encounter in most reports: Counts Per Minute (CPM), MicroSieverts (µSv), and Roentgen (R).
Counts Per Minute (CPM)
Counts Per Minute (CPM) represents the number of radiation events the Geiger counter detects in one minute. This metric serves as an immediate, straightforward indicator of radiation levels. Importantly, it’s often the first marker that users reference when gauging safety levels.
The beauty of CPM lies in its simplicity. For a novice user, CPM offers an easy threshold to monitor; a spike in numbers may indicate caution. However, it’s crucial to remember that a high CPM doesn't automatically correlate to harmful exposure levels. It simply reflects activity. The unit also lacks consideration for the type of radiation, making it less comprehensive than other metrics.
MicroSieverts (µSv)
In terms of health risk, MicroSieverts (µSv) offers a critical perspective. While CPM tells you about radiation activity, µSv quantifies the biological effect of that exposure. It’s a measure of dose that considers the potential health risks associated with exposure to different radiation types.
This unit adds significant value in contexts such as occupational safety where understanding the impact over time is essential. For instance, workers in nuclear facilities might be more concerned with µSv readings than CPM because it provides insight into exposure limits. Despite its usefulness, the conversion from CPM to µSv isn’t straightforward and can vary based on the type of radiation, meaning users need a solid understanding of their specific measurement environment to interpret this correctly.
Roentgen (R)
Roentgen (R) is another measurement that might crop up and usually pertains to gamma and X-ray radiation. It’s noteworthy in historical contexts for being one of the earliest units utilized in radiation measurement. However, this legacy comes with complications – Roentgen does not translate universally to other radiation forms as effectively as µSv does, meaning its relevance might be limited in certain scenarios.
Laboratories monitoring gamma emissions might value Roentgen over CPM for its specificity, yet it’s largely being overshadowed by more contemporary units today. The downside of using Roentgen is that its applicability can seem less clear in mixed radiation environments.
Contextual Factors Influencing Readings
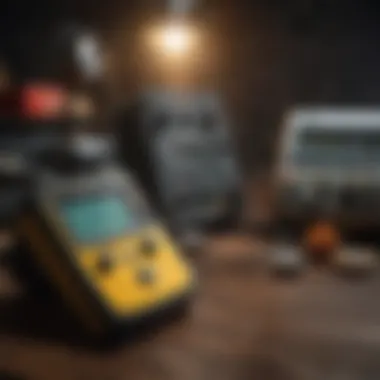
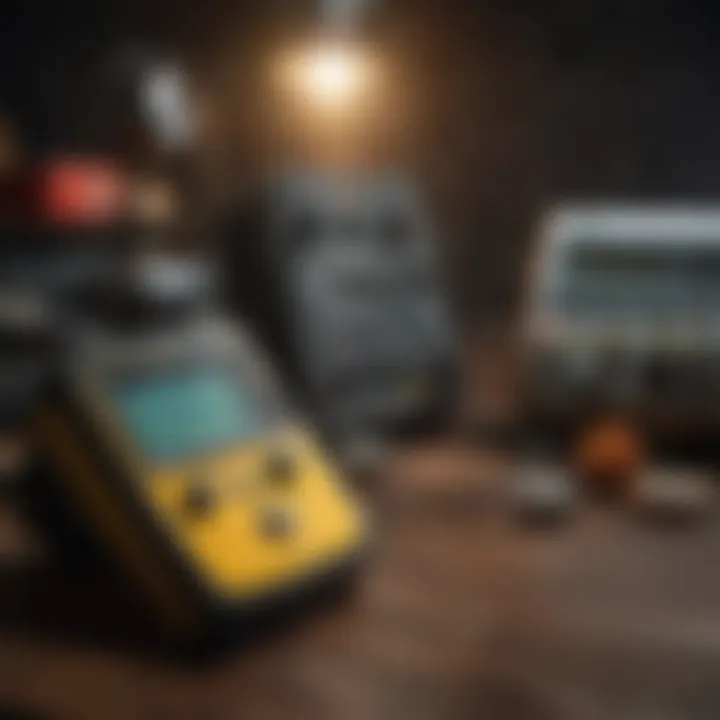
Radiation readings don't occur in a vacuum. Environmental factors play a crucial role in shaping the readings you see on your Geiger counter. Recognizing these factors is key for accurate interpretation.
Environmental Background Radiation
Environmental background radiation reflects the natural ionizing radiation present in our surroundings. This includes cosmic rays, terrestrial radiation from the Earth, and even radon in the air. It’s essential to understand how this background can influence Geiger counter readings, as it can create a baseline level that you must account for.
Failing to recognize background radiation might lead the user to erroneously conclude that a specific area is heavily contaminated when they’re merely detecting normal, background radiation levels. It’s crucial to be cognizant of this when evaluating readings, particularly in different locations.
Calibration Variations
Calibration variations can significantly impact the accuracy of your readings. Each Geiger counter needs to be calibrated periodically to ensure it conforms to standard measurement norms. Variations in calibration can lead to discrepancies that might mislead users into thinking they are exposed to higher or lower levels of radiation than what is truly the case.
For example, if a Geiger counter is calibrated on a specific isotope but later used in an environment with a different radiation type, readings might be imprecise. Being aware of how recent calibration has been conducted and the specific isotope used is critical for accurate readings.
Calibration and Maintenance
Calibrating and maintaining a Geiger counter is not merely a recommended practice; it stands as a cornerstone for achieving accurate and reliable readings. As with any precision instrument, calibration ensures that the Geiger counter functions optimally, providing correct measurements to help users make informed decisions about radiation levels around them. A well-calibrated counter can mean the difference between understanding a safe environment and inadvertently exposing oneself to harmful radiation.
Importance of Calibration
Calibration is fundamental in ensuring the reliability of a Geiger counter. It is the process of comparing the instrument’s measurements against a known standard, allowing any discrepancies to be corrected. In this context, importance can be summarized through several key points:
- Accuracy: Calibration directly impacts the accuracy of readings. An incorrectly calibrated counter may yield misleading information, leading potentially to overestimation of radiation risks.
- Standardization: Regular calibration allows for standard measurement units which is crucial when comparing data across different environments or over time.
- Regulatory Compliance: In many fields, especially healthcare and nuclear industries, compliance with safety regulations is necessary. Proper calibration is often mandated and is critical for legal and operational purposes.
Calibration Procedures
Calibration procedures typically fall into two categories: field calibration and laboratory calibration. Both serve unique purposes and can significantly impact the outcome of radiation monitoring efforts.
Field Calibration
Field calibration involves taking the Geiger counter to the location where it is used, allowing it to be calibrated in its actual working environment. One major benefit of this approach is that it considers the local background radiation which can vary due to environmental factors.
- Key Characteristic: Field calibration focuses on practical use, ensuring the instrument performs as expected under real conditions.
- Benefit: This method provides immediate data, avoiding the need for lengthy laboratory analysis. Users can quickly adjust their devices based on real-time readings.
- Unique Feature: Field calibration takes into account any nearby sources of radiation that might affect readings, thus delivering a more holistic assessment of an instrument's accuracy right where it counts.
- Advantage/Disadvantage: While this may provide quicker results, environmental conditions can introduce variables not present in controlled settings, possibly complicating accuracy assessments.
Laboratory Calibration
Laboratory calibration, on the other hand, typically involves highly controlled conditions where the Geiger counter is tested against certified radiation sources. This approach offers a layer of precision that field calibration may lack.
- Key Characteristic: Laboratory calibration benefits from an environment free of variables, ensuring a high level of accuracy in measurements.
- Benefit: It allows for detailed, rigorous checks against standards set by regulatory bodies, ensuring compliance and potentially reducing liability in professional settings.
- Unique Feature: The use of controlled radiation sources provides a baseline against which the counter's performance can be meticulously measured.
- Advantage/Disadvantage: The main downside is the need to remove instruments from their operational environments, which might not capture real-world variances, making it less ideal for immediate field use.
Routine Maintenance Practices
Beyond calibration, routine maintenance is essential for prolonging the life of the device and ensuring its accuracy. Some practical maintenance tips include:
- Regular Battery Checks: Ensure batteries are functioning well. Low power can disrupt readings and calibration processes.
- Physical Inspections: Look for signs of wear and tear, such as cracks in the casing or damage to cables.
- Documentation: Keep a log of maintenance performed, including calibration dates and findings to track changes over time.
By understanding and implementing these calibration and maintenance principles, users can guarantee the reliability of their Geiger counters, thus empowering them to better manage the challenges that come with ensuring safety around radiation.
Safety Considerations
When working with Geiger counters, comprehending the nuances of safety is paramount. The readings from these devices can vary widely and pose significant risks, especially in contaminated environments. Therefore, understanding safety considerations not only safeguards individuals but also enhances the credibility of the measurements being taken. The implications of miscalculating radiation hazards can range from short-term inconveniences to severe long-term health consequences.
Understanding Radiation Risks
Radiation exposure is often misunderstood. Many believe that low levels of radiation present no threat; however, cumulative exposure can lead to serious health issues. The potential risks are tied to the type of radiation, duration of exposure, and individual susceptibility. For instance, excess exposure to alpha particles can be harmful if ingested or inhaled, while gamma rays penetrate deeply and may cause damage to internal organs.
Recognizing this reality emphasizes the importance of accurate monitoring and understanding Geiger counter readings. With this knowledge, individuals can make informed decisions about when to take protective actions.
Protective Measures
Taking appropriate protective measures is crucial when interpreting Geiger counter readings. These measures fall into various categories, but two of the most prominent are the Use of Personal Protective Equipment (PPE) and the Distance and Shielding Principles.
Use of Personal Protective Equipment (PPE)
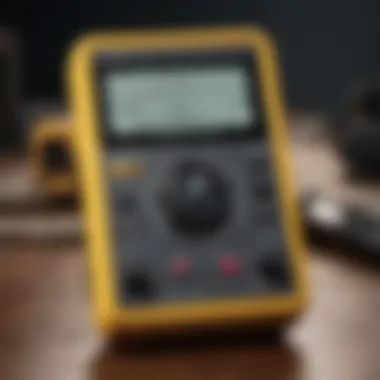
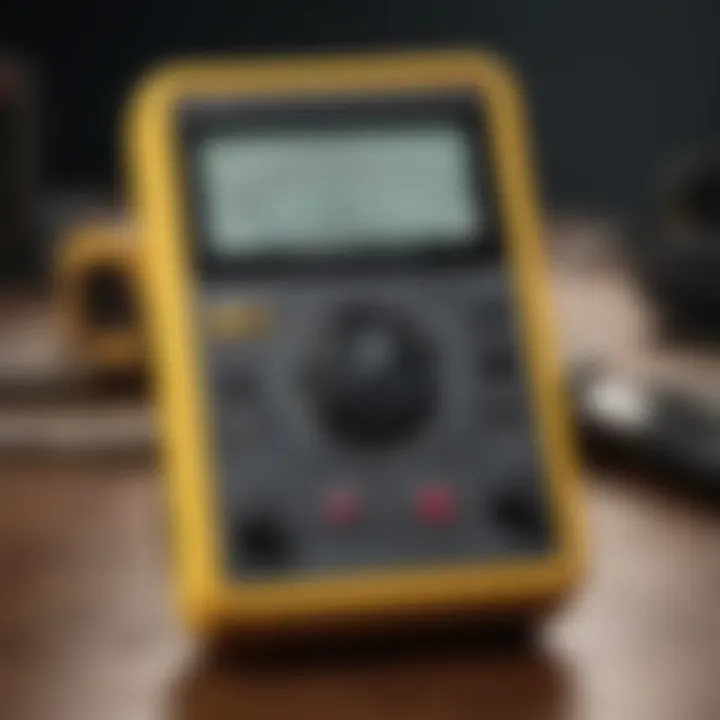
PPE is a frontline defense against radiation exposure. Wearing equipment such as lead aprons, masks, or specialized gloves offers a tangible barrier between the radiation source and the body. One key characteristic of PPE is its ability to reduce radiation dosages significantly. This makes it a popular choice for professionals in environments where radiation levels may fluctuate unexpectedly.
The unique feature of PPE is that it does not only offer protection against ionizing radiation but also provides a sense of safety and confidence to the user. For example, a radiologic technologist wearing appropriate PPE can carry out procedures with greater assurance. However, it is important to note that while PPE is beneficial, it must be properly fitted and maintained to be effective.
Distance and Shielding Principles
Distance and shielding form another crucial protective measure when dealing with radiation. The principle is simple: increasing the distance from a radiation source reduces exposure. This characteristic is not only intuitive but also scientifically backed. The further one is from a radiation source, the lower the radiation dose received, which is why it remains a fundamental concept in radiation safety practices.
Furthermore, shielding involves using materials to block or reduce radiation. For instance, lead is often utilized due to its high density. The unique feature of this principle is the concept of inverse square law, which tells us that doubling the distance from the source results in a fourfold decrease in exposure. Despite its advantages, the immediacy of needing to move away from a source may not always be practical in every situation, highlighting the need for comprehensive safety protocols.
Common Misinterpretations
Understanding how to read a Geiger counter is one thing, but it’s another kettle of fish entirely when it comes to interpreting those readings correctly. Many users, from students to professionals, often fall into the trap of misinterpreting the data presented by this sensitive instrument. This section sheds light on some common pitfalls that can obscure the reality of radiation measurement, helping users avoid unnecessary alarm or confusion. Successful interpretation relies on a solid grasp of these misinterpretations, ensuring that readings serve their intended purpose effectively.
Overestimating Radiation Levels
One major area where misinterpretations creep in is overestimating radiation levels. It’s easy for someone new to using a Geiger counter to see a reading that is higher than expected and jump to conclusions. Perhaps they’ve read an article on radiation dangers and instantly assume they’re in hot water. However, readings can fluctuate due to various factors, including natural background radiation or even the device's sensitivity settings.
"Understanding context is essential when interpreting radiation levels."
To address this, consider the following:
- Background Radiation: Every environment has a baseline level of radiation due to cosmic rays, radon gas, and other natural sources. It’s crucial to know what constitutes a normal reading in your area before panicking over seemingly elevated levels.
- Device Calibration: The calibration and type of Geiger counter can play a big role in readings. A device that hasn't been calibrated properly might give false readings, leading to overestimation of radiation levels.
- Type of Radiation Detected: Not all radiation is harmful. For instance, alpha particles, while easily detectable, require different considerations than gamma rays, which penetrate more deeply and pose higher risks.
Understanding these elements helps to put the readings into perspective.
Misreading Instrumental Faults
Misinterpreting the signs of a malfunctioning Geiger counter can lead you on a wild goose chase. A higher-than-normal reading could be mistaken for a radiation spike when, in fact, it’s just an indicator that your instrument is faulty. Knowing how to spot these issues is key.
When a Geiger counter behaves unexpectedly, here are a few critical aspects to examine:
- Battery Issues: Low batteries can cause erratic readings. If your device starts acting up, check the power source before assuming the worst.
- Mechanical Damage: Look out for visible signs of wear and tear. A device that’s fallen or been knocked around may not provide accurate readings, leading to misinterpretation.
- Environmental Interference: Certain conditions, like heavy electronic equipment nearby, can interfere with the detection process. This isn't a failure of the Geiger counter but rather an external factor affecting performance.
It's vital to conduct routine checks and understand the machine's specifications to differentiate between true readings and instrumental faults.
Applications of Geiger Counters
Geiger counters are crucial tools widely used across various fields, highlighting their versatility and importance. This section explores how these instruments serve specific purposes in environmental monitoring, healthcare, and the nuclear industry. Understanding these applications will shed light on the practical aspects of radiation measurement and how it affects our daily lives.
In Environmental Monitoring
Environmental monitoring is one of the most significant applications of Geiger counters. These devices help gauge the level of radiation present in various ecosystems. Monitoring radiation levels is vital in regions near nuclear power plants or areas with historical nuclear activity, like Hiroshima or Chernobyl.
Using Geiger counters in this context is paramount because of the potential hazards posed by unexpected radiation leaks or contamination. Here are several points to consider:
- Baseline Measurement: Regular readings establish a baseline of normal radiation levels, enabling quick response to any abnormal spikes.
- Wildlife Impact: Monitoring helps assess radiation's long-term effects on wildlife and vegetation in affected environments.
- Public Safety: By reporting radiation levels, these tools inform and protect communities living in close proximity to radiation sources.
The data collected through environmental monitoring can be essential for researchers and policymakers alike, ensuring that both humans and the environment remain safe and protected from radiation exposure.
In Healthcare
Geiger counters play a vital role in healthcare, particularly in medical diagnostics and treatment procedures. Physicians use these devices mainly to monitor and manage radiation used in diagnostic imaging and cancer therapies.
Key Points in Healthcare Applications:
- Radiation Therapy: In treating diseases like cancer, knowing the exact dosage of radiation delivered to a patient is crucial. Geiger counters ensure that the prescribed amount is adhered to, enhancing treatment effectiveness while minimizing injury to healthy tissue.
- Safety Protocols: Healthcare facilities apply stringent safety measures when using radioactive materials. Regular monitoring ensures that staff and patients are not subjected to excessive radiation exposure.
- Screening for Contamination: After certain procedures, healthcare providers often use Geiger counters to verify that there’s no residual contamination in the surrounding area.
These applications help safeguard the well-being of patients and healthcare professionals in environments where radiation is prevalent.
In Nuclear Industry
In the nuclear industry, Geiger counters are indispensable for ensuring safety and compliance with regulations. These devices are used extensively in various facets of nuclear power generation and waste management.
Several important roles include:
- Radiation Surveillance: Geiger counters help monitor radiation levels within nuclear facilities. Regular checks can prevent leaks of radioactive materials that can have severe consequences.
- Worker Safety Monitoring: In environments where radiation is a constant factor, these instruments are crucial in protecting workers. Continuous monitoring enhances safety protocols and informs staff when they may need to vacate zones with high radiation exposure.
- Emergency Response: In response to accidents or unplanned events, rapid radiation assessment is necessary. Geiger counters provide real-time data allowing for quick decision-making in containment or evacuation scenarios.
The application of Geiger counters in the nuclear industry illustrates their critical role in maintaining safety standards while supporting efficient operations.
Understanding the specific applications of Geiger counters not only highlights their importance but also underscores the broader implications for public and environmental health.