Understanding the Human Genome: Structure and Implications
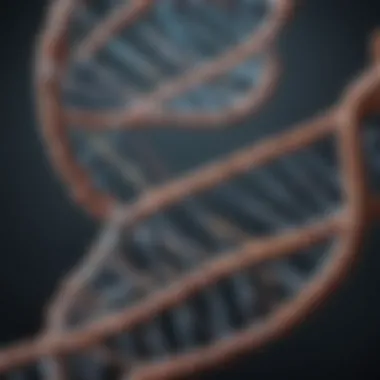
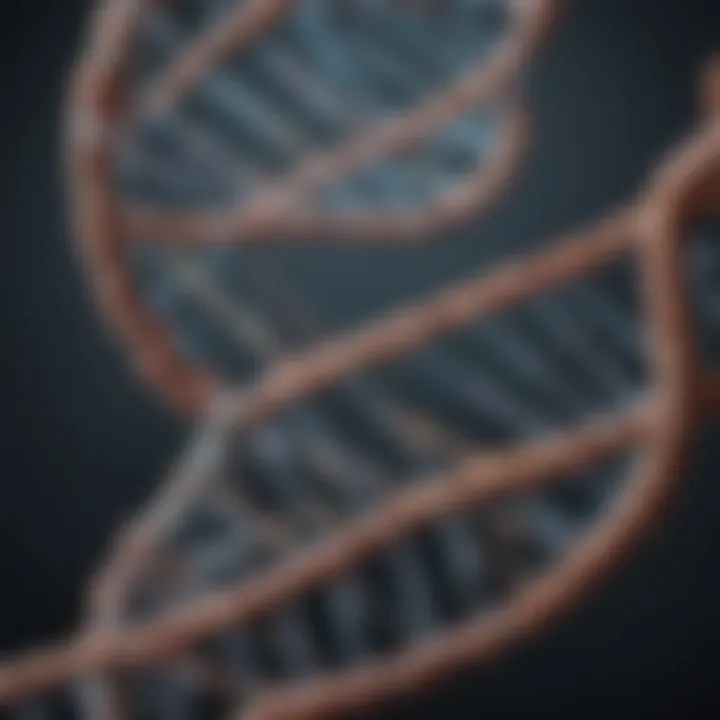
Intro
The human genome represents a fundamental aspect of biology, containing the complete set of inherited information within each human cell. Understanding this intricate blueprint is essential for various fields such as genetics, medicine, and anthropology. Recent advancements in genomic science have unlocked new dimensions of knowledge, providing insights that could transform our comprehensive view of health and disease.
In this article, we aim to dissect the essence of the human genome: examining its structure and components, while also reflecting on the implications it harbors for numerous domains of study. As we navigate through the genome, we will uncover details that are central to grasping its significance in the broader context of human existence.
Research Context
Background and Rationale
The exploration of the human genome is rooted in a rich history of scientific inquiry. Genetic research has evolved significantly since the discovery of DNA's structure by James Watson and Francis Crick in 1953. The realization that DNA is the foundational molecule of life prompted further studies into how genes and genomes dictate biological functions. With the completion of the Human Genome Project in 2003, which mapped the entire sequence of human DNA, researchers gained powerful tools for understanding the genetic underpinnings of human traits and diseases.
The study of the human genome is not merely an academic pursuit. It holds practical implications for fields such as personalized medicine, where genetic profiles can guide treatment strategies. Understanding genetic variations can also lead to prevention strategies in public health, providing insights into disease susceptibility among populations.
Literature Review
Existing literature on the human genome covers a wide range of topics, including but not limited to the identification of genes, their functions, and the implications of genetic variations. Extensive reviews highlight the relationship between genomics and gene expression as well as the impact of epigenetics, which looks at how environmental factors alter gene activity without modifying the DNA sequence itself.
Moreover, advancements in CRISPR technology and gene editing tools have opened new avenues, allowing scientists to manipulate genes with precision. This literature underscores the rapid pace of genomic research and its evolving nature, creating a continuous need for informed discussions and updated interpretations of findings.
The relevance of genomic understanding continues to expand, proving vital for students, researchers, and healthcare providers aiming to bridge scientific knowledge with practical applications. As such, this article intends to synthesize current understanding, aiming for a comprehensive overview of the human genome and its implications for the future.
Definition of the Human Genome
The concept of the human genome is of utmost significance in genetics. The human genome encompasses the complete set of genetic information that is present in humans. It consists of over three billion DNA base pairs organized into structures called chromosomes. Understanding the human genome is crucial as it provides the foundational knowledge necessary for various scientific fields, from medicine to anthropology.
The study of the human genome allows researchers to decode genetic instructions that dictate biological processes. It helps in identifying genetic disorders, understanding human evolution, and improving medical treatments. Moreover, it has critical implications for personalized medicine, where treatments can be tailored based on a person's genetic makeup.
In essence, the human genome serves as a blueprint for human life and understanding it can unlock numerous biological mysteries. Thus, a comprehensive comprehension of its definition and implications is essential.
Overview of Genomic Terminology
In genomic studies, terminology plays a vital role. DNA, or deoxyribonucleic acid, is the molecule that contains the genetic instructions. It is made up of sequences of nucleotides, which are the building blocks of the genome. These sequences vary among individuals, leading to genetic diversity.
Genes are segments of DNA that code for proteins. They are the functional units, carrying instructions for various cellular functions. The human genome contains about 20,000 to 25,000 genes.
Chromosomes are structures within cells that organize DNA. Humans have 23 pairs of chromosomes, inheriting one set from each parent. Each chromosome holds many genes and regulatory elements, and their organization is crucial for proper gene expression.
Other important terms include genotype, which refers to the genetic constitution of an individual, and phenotype, which is the observable expression of the genotype in the form of physical traits and behaviors. Understanding these terms is essential for grasping the complexities of genomic research.
Historical Context of Genomic Research
Genomic research has a rich history that dates back over a century. The discovery of DNA as the carrier of genetic information was a pivotal moment. In the early 20th century, scientists like Gregor Mendel laid the groundwork for understanding heredity.
In 1953, James Watson and Francis Crick proposed the double-helix structure of DNA. This discovery opened a new era in molecular biology. The Human Genome Project, initiated in 1990 and completed in 2003, aimed to map all the genes in the human genome. It was a landmark achievement that provided a wealth of information, fundamentally changing genetics and medicine.
Since then, research has expanded exponentially. Advances in technology, like next-generation sequencing, have made it possible to study the genome in depth. The implications of these advancements reach beyond the lab, impacting ethics, medicine, and our understanding of human existence itself.
Structural Components of the Human Genome
The structural components of the human genome are foundational to understanding genetic information. Each element carries a significance that contributes to the overall function of our biology. Analyzing these components provides insights into how genetic traits are inherited, how diseases manifest and how genomic technologies advance medical practices. This section will cover DNA, genes, and chromosomes, each a crucial puzzle piece in the genomic landscape.
DNA: The Primary Molecule
DNA, or deoxyribonucleic acid, is the molecule that stores genetic instructions. Its double-helix structure was first described in 1953 by James Watson and Francis Crick. This structure allows DNA to replicate accurately during cell division, ensuring that genetic information is passed on.
The DNA molecule is composed of smaller units called nucleotides, each containing a phosphate group, a sugar, and a nitrogenous base. The arrangement of these basesāadenine, thymine, cytosine, and guanineādetermines the genetic code. DNA is not only essential for heredity but also crucial for the synthesis of proteins, which perform most essential functions in living organisms.
Additionally, DNA serves as a template for RNA production through a process called transcription. RNA then plays a vital role in protein synthesis, emphasizing the importance of DNA in cellular function.
Genes and Their Functions
Genes are segments of DNA that contain the instructions for making proteins. Each gene has a specific location on a chromosome and can vary in size. The average human gene consists of around 1,000 base pairs, but some can extend to millions of base pairs.
The functions of genes are vast and varied. They influence characteristics such as physical traits, metabolism, and susceptibility to diseases. Genes can be classified into two broad categories: protein-coding genes and non-coding genes.
- Protein-coding genes: These genes are translated into proteins that carry out various functions in the body.
- Non-coding genes: These genes do not code for proteins but have regulatory or structural roles that are equally significant.
Mutations in genes can lead to changes in protein function, which may cause or contribute to various diseases. Understanding these gene functions is fundamental in fields like medical genetics and biotechnology.
Chromosomes: Organization of Genetic Material
Chromosomes are structures that organize and compact DNA within the cell nucleus. In humans, there are 23 pairs of chromosomes, with one set inherited from each parent. Each chromosome contains numerous genes, akin to books in a library, where each book holds information about the traits and functions of an organism.
The first 22 pairs are called autosomes, and the last pair is the sex chromosomes (XX in females and XY in males). Chromosomes undergo replication during cell division, ensuring that genetic material is correctly distributed to daughter cells. This organization is critical because it allows for the correct expression of genes and maintains genomic stability.
In summary, understanding the structural components of the human genome lays the groundwork for further exploration into genomic research. With the knowledge of DNA, genes, and chromosomes, one can better appreciate the complexities of heredity and the implications for health and disease.
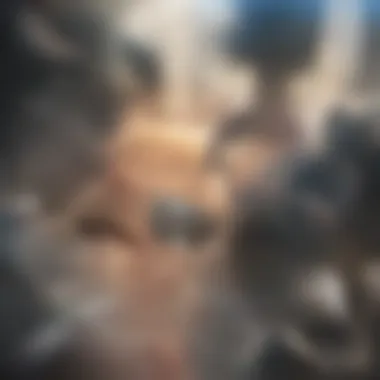
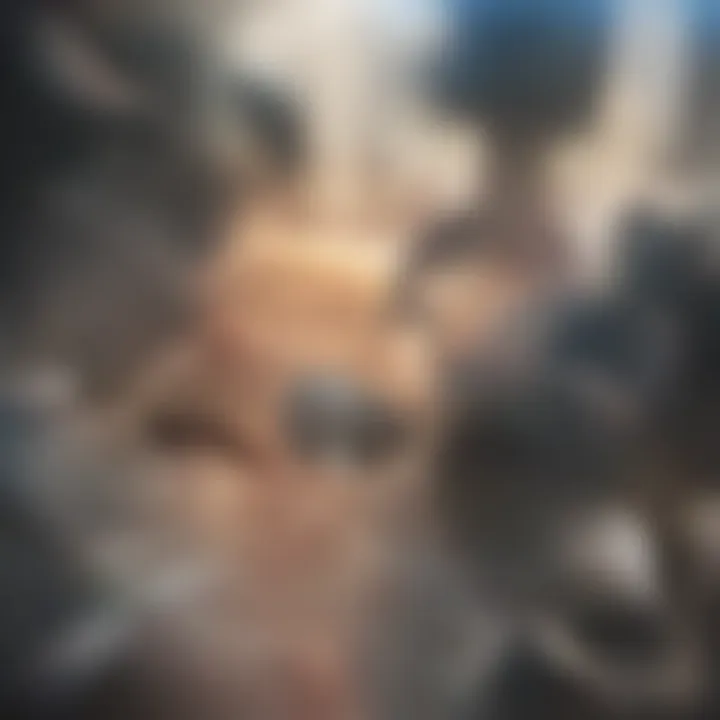
"Understanding the genomic structure is crucial for any advances in genetic research and personalized medicine."
This knowledge is vital for future advancements in medical therapies, biotechnology, and evolutionary studies.
Genomic Variability in Human Populations
Genomic variability is crucial in understanding the human genome. It helps outline how different genetic factors contribute to various traits and predispositions among individuals. This subsection examines key elements of genomic variability such as Single Nucleotide Polymorphisms (SNPs), Copy Number Variations (CNVs), and the broader significance of genomic diversity as a whole. By analyzing these components, we can better appreciate how they influence health, disease, and overall population dynamics.
Single Nucleotide Polymorphisms (SNPs)
Single Nucleotide Polymorphisms, or SNPs, represent the most common type of genetic variation among people. Each SNP is a difference in a single nucleotide ā the building blocks of DNA ā occurring in at least 1% of the population. They play a pivotal role in personalizing medicine since SNPs can affect an individual's response to drugs, susceptibility to environmental factors, and risk for diseases.
For instance, a specific SNP in the gene TPMT can influence how individuals metabolize thiopurine drugs, which are used to treat certain cancers and autoimmune diseases. Understanding these variations helps clinicians tailor treatments that are most effective for each patient. In summary, SNPs are more than just trivial differences; they highlight the intricate genetic landscape that affects our health and wellness.
Copy Number Variations (CNVs)
Copy Number Variations (CNVs) are larger-scale variations in the genome. They involve duplications or deletions of sections of the DNA that can encompass one or more genes. CNVs contribute to genomic diversity and have been linked to various phenotypic outcomes, from normal variation to predispositions for diseases.
Research indicates that CNVs may play a role in complex diseases such as schizophrenia, autism, and certain cancers. In addition, CNVs can affect gene expression levels, influencing traits in populations. The complexity of these variations requires comprehensive methodologies to ascertain the full extent of their influence on health and disease outcomes.
The Role of Genomic Diversity
The role of genomic diversity cannot be overstated. It is the basis for the uniqueness of individuals, impacting not only physical traits but also the overall adaptability of populations. Genetic diversity is fundamental for a species' survival. It enables human populations to adapt to changing environments, resist diseases, and prevent the detrimental effects of inbreeding.
In addition, studying genomic diversity highlights the historical migrations and evolutionary pressures faced by humans. Identifying variations across different populations can provide insights into human history and migration patterns, further contributing to our understanding of genetics.
"The study of genomic variability presents an unprecedented opportunity to scrutinize the foundation of our speciesā biological and cultural diversity."
Technological Advances in Genomic Research
Technological advances in genomic research have fundamentally transformed our understanding of the human genome. These developments have unlocked new avenues for exploration, making it possible to analyze genetic information with unprecedented precision. As such, the implications extend from personalized medicine to evolutionary biology, significantly impacting multiple fields.
Key elements of these advances include the methodologies adopted for sequencing genomes, the tools used for data analysis, and the technologies leveraged for genome editing.
Next-Generation Sequencing (NGS)
Next-Generation Sequencing (NGS) represents a remarkable leap in the way genetic data is obtained. Traditional sequencing methods were relatively slow and limited in scope, often rendering the process labor-intensive and expensive. In contrast, NGS facilitates massively parallel sequencing of multiple DNA strands, drastically increasing throughput.
The benefits of NGS include:
- High Accuracy: Enhanced precision in reading sequences allows for better identification of genetic variants.
- Speed: The rapid processing of vast amounts of data leads to quicker results, which is crucial for time-sensitive research and clinical settings.
- Cost-effectiveness: Economies of scale have made genomic sequencing accessible, enabling large-scale studies that were unaffordable previously.
These factors enable researchers to characterize the genome in ways that were once deemed impossible, allowing for deeper insights into genetic disorders and variations in populations.
Bioinformatics in Genomics
Bioinformatics plays a crucial role in the landscape of genomic research. With the voluminous data generated by NGS, it becomes imperative to have sophisticated tools for storage, analysis, and interpretation of genomic data. Bioinformatics combines biology, computer science, and mathematics to create methods that can analyze complex biological data.
The significance of bioinformatics can be identified through several points:
- Data Management: Effective handling of large datasets ensures that valuable findings are not lost.
- Analytical Tools: Algorithms and software applications help in identifying correlations, such as disease associations with specific genes.
- Predictive Analytics: Machine learning models can predict gene functions and interactions, offering a glimpse into biological processes.
As bioinformatics continues to evolve, it also enhances our understanding of genomic elements and their roles in health and disease.
CRISPR and Genome Editing
CRISPR technology has stirred considerable interest and debate in genomic research. This revolutionary tool allows for precise editing of the genetic code. The implications of CRISPR are extensive, making it a focus of contemporary genetic studies.
Key aspects of CRISPR include:
- Versatility: Ability to edit genomes across a wide range of organisms.
- Precision: Targeting specific DNA sequences minimizes the risk of unintended mutations, known as off-target effects.
- Wide Applicability: From agriculture to medicine, CRISPR has the potential to contribute to various sectors, including disease modeling and treatment strategies for genetic disorders.
However, the ethical considerations surrounding gene editing, especially in humans, cannot be overlooked. The intersection of technology and morality presents a continuous challenge to scientists and society alike.
Technological advances in genomics do not just enhance our technical capabilities; they reshape our understanding of life itself.
In summary, technological advances in genomic research, marked by initiatives like Next-Generation Sequencing, bioinformatics integration, and CRISPR innovation, are creating a transformative landscape. These tools not only aid in deciphering the human genome but also inform future strategies for health and disease management.
Implications of Human Genomics
The implications of human genomics are vast and intricate, offering insights that affect various sectors such as medicine, ethics, and technology. Understanding these implications is crucial for harnessing the full potential of genomic research. This section addresses several key elements that outline the value and considerations necessary in this field.
Genomics and Personalized Medicine
Personalized medicine represents one of the most significant advancements stemming from human genomic studies. It involves tailoring medical treatment to the individual characteristics of each patient. Genomic data allows healthcare providers to determine the most effective therapies based on a personās genetic makeup. For instance, certain cancer treatments are now matched to specific genetic mutations present in tumors, improving outcomes while minimizing adverse effects. This shift towards a more individualized approach elevates patient care and has the potential to revolutionize how diseases are diagnosed and treated.
Key aspects include:
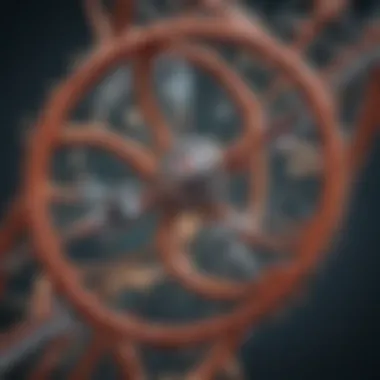
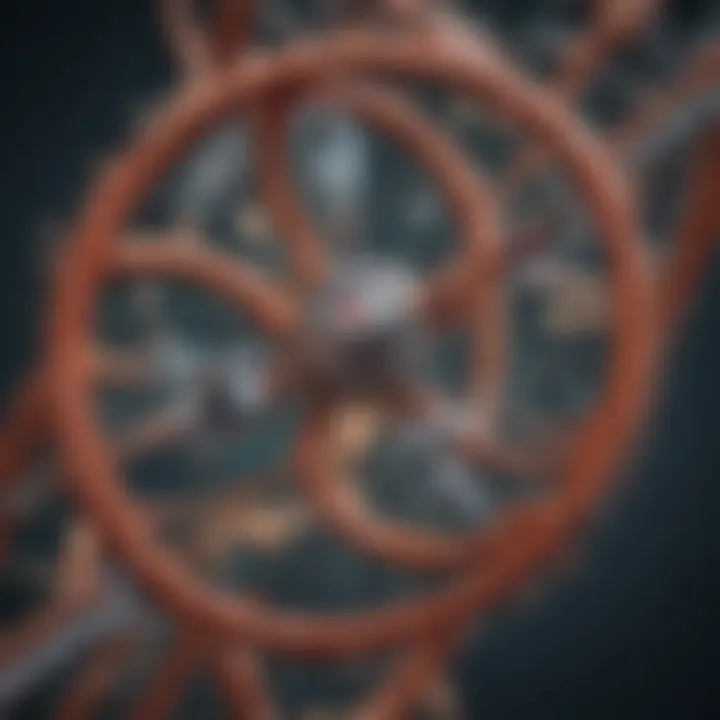
- Targeted therapies: Medications designed to target specific genetic abnormalities.
- Genetic testing: Tools to predict individual responses to treatments.
- Preventative steps: Strategies to reduce the risk of developing diseases based on genetic predispositions.
Ethical Considerations in Genomic Research
The rise of genomic technologies also raises numerous ethical issues that must be navigated with care. Genomic data is sensitive information, and its misuse can lead to discrimination, stigmatization, or privacy violations. Considerations such as the consent for genetic testing and data sharing, the potential for genetic discrimination by employers or insurers, and the implications of gene editing present critical discussions within the scientific community and society at large.
Some ethical challenges include:
- Informed consent: Ensuring that participants fully understand the risks and benefits before providing genetic data.
- Data ownership: Questions about who controls and accesses genetic information.
- Equity in access to technologies: Addressing disparities in who benefits from advancements in genomics.
As genomic technologies advance, ethical guidelines must evolve to protect individuals and address these complex issues.
The Future of Genetic Technologies
Looking forward, the future of genetic technologies holds significant promise alongside considerable challenges. Innovations in artificial intelligence are set to enhance genomic research, allowing quicker data analysis and improved predictive models. These advancements have the potential to facilitate breakthroughs in understanding complex diseases, leading to more efficient drug discovery processes.
Furthermore, the scope of gene editing, exemplified by CRISPR technology, is expanding rapidly. Future developments may allow for highly precise editing of genes to prevent inherited diseases or tailor crops for better yield and resilience.
Key predictions include:
- Increased integration of AI: Streamlining the research process and producing more accurate genomic insights.
- Expansion of gene therapies: Treating genetic disorders at their source.
- New ethical frameworks: Developing guidelines to address emerging concerns as technology evolves.
The potential benefits of genomic research stand to shape multiple aspects of human life, but the ethical and practical considerations must be continually assessed to ensure responsible use.
These various implications underline why understanding human genomics is essential not only for scientists but also for policymakers, ethicists, and the public. They highlight the dynamic intersection of genetic science with everyday life, shaping the way we approach health, identity, and social responsibility.
The Human Genome Project: A Milestone in Genomics
The Human Genome Project (HGP) marks a pivotal moment in the field of genomics. Launched in 1990 and completed in 2003, this monumental collaboration aimed to sequence the entire human genome ā the complete set of DNA that defines human biology. The HGP has profoundly influenced not only genetic research but also medicine, anthropology, and ethics surrounding genetic information. This project was ambitious, involving hundreds of researchers from various countries and institutions, symbolizing a collective effort to map human DNA.
Goals of the Project
The primary goals of the Human Genome Project were clear and focused.
- Sequencing the Human Genome: The foremost aim was to determine the nucleotide sequence of the entire human genome. This includes identifying the approximately 20,000-25,000 human genes.
- Mapping Genetic Variation: Understanding genetic differences among individuals was crucial. The project aimed to create a detailed map of human genetic variation, which includes identifying Single Nucleotide Polymorphisms (SNPs).
- Enhancing Technological Capabilities: Another goal was to develop technologies that could improve DNA sequencing and analysis. These advancements would benefit future genetic research.
- Facilitating Data Availability: The HGP sought to ensure that genomic data generated would be accessible to researchers worldwide, promoting transparency and collaboration in genomic studies.
Key Findings and Contributions
The Human Genome Project yielded several key findings that have shaped the future of genetics.
- Complete Sequence of the Human Genome: The HGP succeeded in providing a reference sequence for the entire human genome, which serves as a baseline for understanding human genetics.
- Identification of Genes and Their Functions: It discovered many human genes, their locations, and functions, enriching the knowledge base about genetic disorders and biological processes.
- Establishment of Genetic Variability: The project highlighted the extent of genetic variation among human populations, enhancing our understanding of evolutionary biology and population genetics.
- Technological Innovations: The methodologies developed during the HGP have significantly advanced genomic technology, leading to innovations like Next-Generation Sequencing (NGS).
Impact on Modern Genomics
The influence of the Human Genome Project on modern genomics is undeniable.
The findings have laid a foundation for personalized medicine, where treatments can be tailored to an individualās genetic profile. Moreover, the data generated from the HGP has driven research in various fields, such as cancer genomics, pharmacogenomics, and rare genetic disorders.
Additionally, the HGP has sparked discussions about bioethics, particularly in the realm of genetic privacy and data sharing. As more genetic data becomes available, questions arise concerning who has access to this information and how it can be used responsibly.
In essence, the Human Genome Project stands as a landmark achievement in genetics, reshaping research methodologies and ethical considerations, while setting the stage for future innovations in genomics.
Applications of Human Genome Research
The study of the human genome offers a treasure trove of applications that extend far beyond traditional genetic research. Understanding the genome helps in disease prevention and management, agricultural advancements, and forensic science. Each of these applications showcases how valuable genomic research can be to society and various fields. The relevance of this topic is profound, as the implications touch laws, economy, ethics, and more. These applications highlight the collaborative nature of genomics with medicine, agriculture, and law enforcement.
Disease Prevention and Management
The role of genomics in medicine is one of its most significant applications. Researchers use genetic information to identify predispositions to certain diseases, allowing for early interventions. Genomic data helps in tailoring preventive measures based on individual risk factors. This personalization means patients can receive targeted treatments that are more effective and less invasive than standard approaches.
- Genetic Testing: Many conditions, like certain cancers, have genetic markers. Testing can identify these markers, guiding screening decisions.
- Pharmacogenomics: This subfield examines how genes affect a person's response to drugs. In turn, it aids in selecting the most suitable medications for individuals, minimizing adverse effects.
- Treatment Development: Understanding genetic variations paves the way for developing novel therapies. Drugs can be designed to target specific genetic profiles, enhancing treatment effectiveness.
"Genomic research not only changes our approach to existing diseases but also provides a framework for preventing them."
Agricultural Biotechnology
Genomics also plays a crucial role in agriculture. Scientists use genomic tools to enhance crop yield, resilience, and nutritional value. This biotechnology branch focuses on understanding plant genomes and improving traits that can withstand environmental pressures.
Developments include:
- Genetically Modified Organisms (GMOs): These organisms are engineered for specific traits, such as pest resistance or improved growth rates.
- Marker-Assisted Selection: This approach accelerates the breeding process. It uses genetic markers to select for desirable traits in plants and animals more efficiently.
- Disease Resistance Development: Crop varieties with enhanced resistance to diseases ensure food security and reduce reliance on chemical pesticides.
Forensic Science Applications
The integration of genomic science in forensics has transformed investigative processes. DNA profiling based on genomic data enables the identification of individuals with remarkable accuracy. This field uses genetic information for purposes including:
- Criminal Investigations: DNA collected from crime scenes can be matched to suspects or to create profiles of unidentified individuals.
- Paternity Testing: Genetic tests determine biological relationships, aiding in both legal and personal contexts.
- Identification of Remains: In cases where bodies are unrecognizable, DNA analysis assists in identifying victims, providing closure for families.
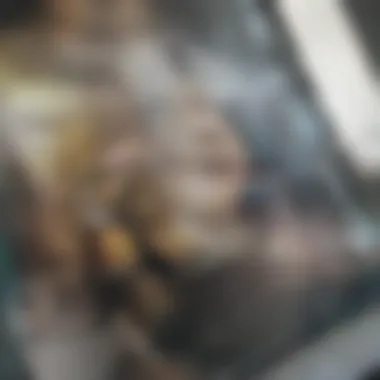
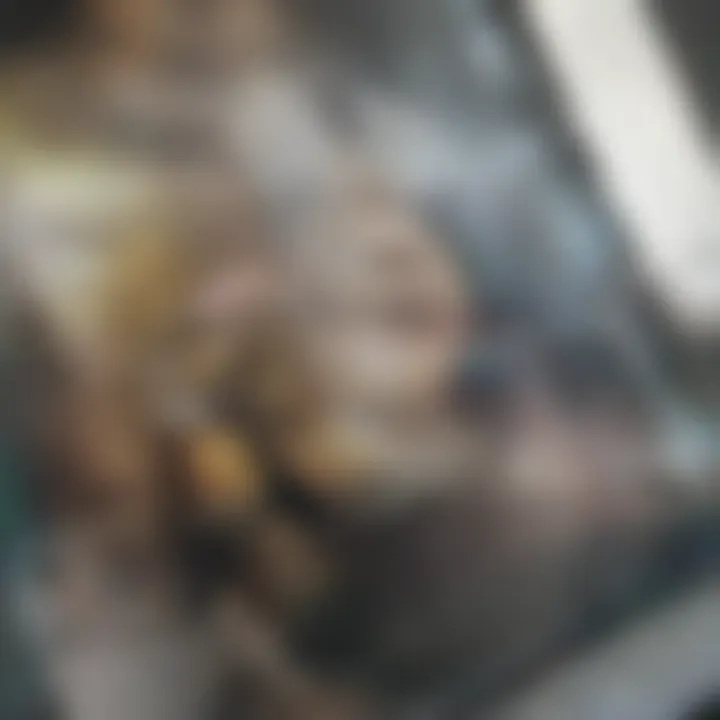
Overall, the applications of human genome research are vast and impactful. They not only enhance our understanding of human biology but also yield practical solutions in healthcare, agriculture, and forensic science. The ongoing advancements promise further development in these fields, holding potential for societal transformation.
Challenges in Human Genomic Studies
Understanding the challenges in human genomic studies is essential for researchers and practitioners alike. The complexities and ethical dimensions of genomic data compel attention to specific aspects that may influence research outcomes and applications. Navigating through these challenges can ultimately shape the direction of genomics as a field and its integration into daily life and healthcare.
Data Privacy and Security
With the rise of genomic research, the importance of data privacy cannot be overstated. Genomic data contains sensitive information that may reveal not just individual traits but also predispositions to various diseases. Therefore, protecting this data is crucial.
- Disclosure Risks: Individuals fear that unauthorized access to their genomic information could lead to discrimination, particularly in insurance and employment.
- Legal Implications: The Human Genome Project established ethical and legal frameworks, yet these laws often vary by country, creating uncertainty in enforcement.
- Technological Solutions: Advanced encryption methods and secure databases should be employed to safeguard genomic data, but these solutions also come with their own costs and limitations.
Interpretation of Genomic Data
The interpretation of genomic data poses significant challenges. Even with robust data, the complexity of genetic interactions is daunting. Variables such as environmental influences and epigenetics complicate direct correlations between genetics and health outcomes.
- Informed Consent Issues: Participants in genomic studies may not fully understand what data interpretation entails, leading to misunderstandings about the implications of their genetic data.
- Expertise Gap: The growing volume of genomic data calls for professionals trained in both genetics and bioinformatics. As demand outpaces supply, quality interpretation can suffer.
- Evolving Knowledge: As research progresses, earlier interpretations might become outdated, necessitating continuous learning and adaptation.
Socioeconomic Barriers to Access
Access to genomic research and its benefits are not evenly distributed. Socioeconomic factors often dictate who can benefit from advancements in genomics, creating disparities in healthcare outcomes.
- Cost of Testing: Genetic testing can be prohibitively expensive, limiting access to those who can afford it. This raises questions about equity in healthcare.
- Awareness and Education: Public understanding of genomics is limited. Campaigns to raise awareness must address these gaps to promote equitable access to genetic advances.
- Infrastructure: In under-resourced areas, the lack of proper technology and trained professionals further exacerbates the divides in access.
"Understanding the challenges in human genomics is not just about science; it involves ethical and social considerations that must be addressed to move forward responsibly."
In summary, while the potential of human genomic studies is vast, it is crucial to navigate the challenges of data privacy, interpretation, and access. Addressing these issues thoughtfully will aid in realizing the full potential of human genomics in a fair and secure manner.
The Role of the Human Genome in Evolutionary Studies
Understanding the human genome plays a critical role in evolutionary studies. It offers insights that help decode the sequence of events that shaped human evolution. By examining genetic similarities and differences, researchers can trace the lineage and migration patterns of ancient populations. This analysis enables scientists to reconstruct evolutionary trees, permitting a better understanding of our shared ancestry with other species.
The significance of the human genome extends to several aspects:
- Genetic Bottlenecks: Studying the variations within the human genome can reveal how populations have responded to historical events, such as natural disasters or significant climate changes, which might have caused genetic bottlenecks.
- Adaptive Evolution: Some genes show signs of adaptation in response to environmental pressures. This can inform us about how humans have evolved to cope with varying climates and diets.
"The human genome is not merely a record of ancestry; it's a genomic fingerprint of our survival strategies across time."
- Health Disparities: Understanding genetic diversity is vital for addressing health issues prevalent in certain populations. Such knowledge can guide public health initiatives and genetic counseling.
Comparative Genomics
Comparative genomics involves comparing the human genome with those of other organisms. This discipline enables insights into evolutionary relationships and the functions of various genes. Scientists utilize whole-genome sequencing to identify conserved sequences across species, permitting them to infer which genes are fundamental for survival and which are recent adaptations.
Key points in comparative genomics include:
- Identifying Homologous Genes: By examining similar genes across different species, researchers can determine which genes have preserved their function throughout evolution.
- Gene Family Expansion: Some gene families expand or contract in different lineages. This can indicate how certain traits evolved in specific environments.
Examples of species often compared to humans include:
- Chimpanzees: Sharing about 98% of our DNA, they provide insights into human-specific traits.
- Neanderthals: Analyzing their genome helps us understand interbreeding events in human evolution.
- Other Mammals: Such comparisons can illuminate the evolution of physiological traits or adaptations.
Tracing Human Ancestry
Tracing human ancestry through the genome relies heavily on analyzing patterns in genetic variation. By studying markers such as mitochondrial DNA and Y-chromosome variations, scientists can reconstruct maternal and paternal lineages.
Several important factors aid in tracing ancestry:
- Mitochondrial DNA Analysis: This genetic material, passed down through mothers, allows researchers to trace matrilineal ancestry. It remains relatively unchanged over generations, which helps determine ancient human migration routes.
- Y-chromosome Studies: The Y-chromosome analysis focuses on paternal lineage, providing alternative perspectives on human ancestry. It reveals male-specific genetic markers since males inherit these from their fathers.
- Ancient Genomes: Sequencing ancient genomes enables direct comparisons with modern humans, helping to clarify the relationships and differences between ancient and contemporary humans.
Future Directions in Human Genomics
The field of human genomics continues to evolve rapidly, paving the way for the development of innovative scientific approaches that could redefine our understanding of genetics. Various facets of research and technology show promise in enhancing personalized medicine and resolving complex biological inquiries. This section explores future directions in human genomics, focusing on its potential advances and implications.
Advancements in Genomic Engineering
Genomic engineering holds a transformational potential in the what we know about genetics. The rise of technologies such as CRISPR-Cas9 enables precise editing of the DNA sequence. This allows scientists to address genetic disorders at their roots. Researchers can potentially correct mutations responsible for diseases like sickle cell anemia or cystic fibrosis.
Considerations for this cutting-edge technology include ethical guidelines. Discussions regarding who can access such technologies and under what conditions are essential. Furthermore, potential unintended consequences of gene editing must also be considered.
The notion of creating 'designer babies' brings a range of ethical dilemmas. Nonetheless, advancements in genomic engineering can significantly contribute to health improvements and disease prevention.
Integration of Artificial Intelligence in Genomics
Artificial Intelligence (AI) is substantially revolutionizing various fields, and genomics is no exception. In the context of genomics, AI can analyze vast datasets far more efficiently than traditional methods. This helps in identifying patterns and correlations within genetic data.
AI algorithms help in predicting disease risk based on genomic information. These predictive insights can lead to early diagnosis and tailored treatment plans. The integration of AI can streamline genomic research, making it more accessible and cost-effective.
Among the challenges faced by AI in genomics is the accuracy of predictions based on models. Data quality and representativeness play crucial roles. Moreover, privacy concerns regarding the handling of personal genetic data pose a challenge. Balancing technological advancement with ethical standards remains paramount.
"The merging of AI and genomics could lead to breakthroughs that were previously thought impossible."
As the fields of genomics and AI continue to converge, researchers and industry professionals must remain vigilant about the implications of their work, ensuring that benefits are realized while minimizing risks and ethical concerns.
Advancing human genomics toward a more comprehensive understanding of health and disease is essential. These future directions highlight the promising potential in genomic engineering and AI, engaging both scientific communities and the public.