Understanding Solar Energy Production and Its Impact
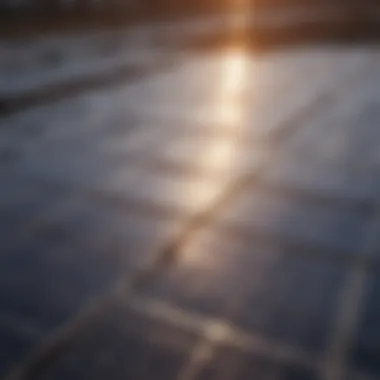
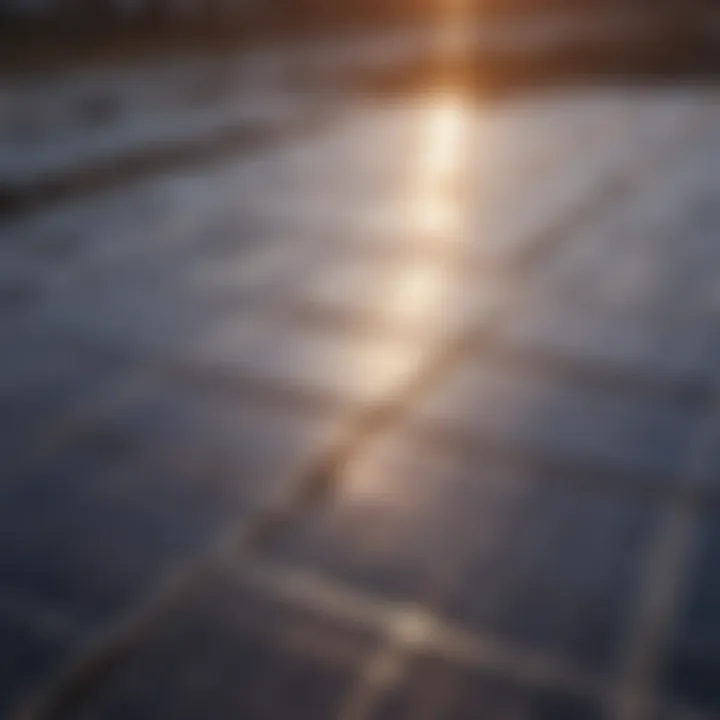
Intro
Solar energy has emerged as a cornerstone in the dialogue about sustainable energy sources. As concerns about climate change mount and fossil fuel resources dwindle, effective harnessing of solar power offers a viable pathway toward energy sustainability. Understanding the intricate details of solar energy production is essential for students, researchers, educators, and professionals who wish to engage critically with this pivotal subject.
The significance of solar energy lies not just in its abundance but also in its potential to generate clean, renewable energy. With technological advancements accelerating over the years, the efficiency of solar energy systems has improved markedly. This article aims to dissect these complexities, enlightening readers on how systems such as photovoltaic and solar thermal harness solar energy effectively.
The following sections will delve into the research context, examining the background and rationale behind solar energy studies, as well as analyzing existing literature. Subsequently, we will discuss the methodologies that inform current research in solar energy production. By presenting a clear narrative, readers will develop a robust understanding of how solar energy operates within broader energy systems and its economic implications, ultimately empowering informed discussions on energy policy.
Preface to Solar Energy
Solar energy forms a pivotal element in the discussion surrounding renewable energy options today. As concerns about fossil fuel dependence and climate change grow, understanding solar energy becomes increasingly critical. Solar energy, harnessed from the sun's radiation, offers a sustainable alternative that can aid in reducing greenhouse gas emissions while providing electricity and heating solutions.
Definition of Solar Energy
Solar energy refers to the radiant light and heat emitted by the sun. It can be captured using a variety of technologies, primarily solar photovoltaic (PV) cells and solar thermal systems. By converting sunlight into usable energy, these technologies play a key role in meeting energy demands worldwide. This energy can then be utilized for generating electricity, heating homes, powering vehicles, and a variety of other applications.
Importance of Solar Energy
The significance of solar energy in today’s energy landscape cannot be overstated. Here are several important factors:
- Environmental Benefits: Unlike traditional energy sources, solar energy production leads to minimal environmental impact. It contributes to reduced air and water pollution, helping to combat climate change.
- Energy Independence: Utilizing solar energy can decrease reliance on imported fossil fuels. Countries equipped with adequate sunlight can harness this resource, fostering local energy production.
- Technological Advancements: Ongoing advancements in solar technology enhance energy efficiency and reduce costs. These improvements make solar energy more accessible and attractive for both consumers and industries.
- Economic Opportunities: The solar industry creates jobs in research, installation, and maintenance. As demand for solar energy increases, so do the employment prospects within this sector.
Understanding solar energy is crucial not just for technological advancement, but also for enabling global efforts towards a more sustainable future.
Basic Principles of Solar Energy Production
The basic principles of solar energy production are fundamental to comprehending how solar technology operates and its potential benefits. At the core, these principles involve the conversion of sunlight into usable energy. This process underpins both photovoltaic and solar thermal systems, serving as a foundation for innovations and applications in sustainable energy. Understanding these principles not only aids in grasping current technologies but also informs future advancements in efficiency and integration with existing energy systems.
Understanding Light and Energy Conversion
Light primarily comes from the sun and is a key player in energy generation. Solar energy production begins with the absorption of sunlight, which consists of particles called photons. When these photons hit a solar cell, they excite electrons, creating a flow of electric current. This concept is often referred to as the photovoltaic effect. The efficiency of this conversion is critical to the performance of solar systems. Factors such as the angle of sunlight, temperature, and the material properties of the solar cells play pivotal roles in determining energy output.
- Photovoltaic Effect: The interaction of sunlight with semiconductor materials forms the basis of energy conversion.
- Efficiency Factors: Angle of incidence, temperature, and the quality of materials can significantly influence conversion efficacy.
Through research, specialists have developed various materials to enhance this energy conversion process. Each material has its unique properties, impacting how effectively it can transform sunlight into electicity.
Solar Spectrum
The solar spectrum encompasses the range of wavelengths emitted by the sun, spanning ultraviolet, visible, and infrared light. Each type of light possesses distinct energy levels. The challenge in solar energy production lies in effectively harnessing this spectrum. Different technologies respond variably to the solar spectrum, affecting their overall efficiency.
- Ultraviolet Light: High energy, but only a small portion reaches the Earth's surface.
- Visible Light: Optimum for photovoltaic cells, as they are designed to absorb this range effectively.
- Infrared Light: Carries significant energy but requires specialized systems for conversion.
Understanding the solar spectrum allows for the enhancement of solar cell designs. By developing materials that can absorb broader wavelengths, researchers aim to increase energy capture from sunlight. Additionally, optimizing solar systems for different light conditions can lead to improved performance in varying climates and locations.
"The solar spectrum is not just about sunlight; it is about how effectively we can convert this energy into usable forms."
In summary, the basic principles of solar energy production, namely the conversion of light into energy and the importance of understanding the solar spectrum, are essential for advancing solar technology and addressing global energy challenges.
Photovoltaic Technology
Photovoltaic technology is a critical area of focus in solar energy production. This technology is at the heart of converting sunlight directly into electricity. It plays a pivotal role in making solar energy a viable alternative to traditional energy sources. With the world moving toward renewable energy, understanding photovoltaic systems is essential for anyone interested in sustainable practices.
Mechanics of Photovoltaic Cells
Photovoltaic cells, often called solar cells, operate based on the photoelectric effect. This process involves the absorption of photons, which prompts electrons to move, creating an electric current. The core components of a photovoltaic cell include semiconductors, generally silicon-based, which are treated to form a positive and a negative layer. When sunlight hits these layers, it stimulates the electrons and generates electricity.
The efficiency of these cells is dependent on factors such as material quality, environmental conditions, and installation angle. Generally, photovoltaic cells are designed to maximize light absorption, ensuring that the most energy possible is captured and converted into usable electricity.
Types of Photovoltaic Cells
Different types of photovoltaic cells exist, each with its unique characteristics and uses.
Monocrystalline Cells
Monocrystalline cells are made from a single crystal structure. These cells are known for their high efficiency and longevity. Their key characteristic is the uniformity of the silicon used, which allows for optimal electron flow. Monocrystalline cells are a popular choice in residential applications due to their aesthetic appeal and space efficiency. However, they tend to be more expensive compared to other types.
Advantages:
- High efficiency rate (up to 22% or more)
- Long lifespan, often exceeding 25 years
Disadvantages:
- Higher initial cost
- Performance can decline in extreme heat
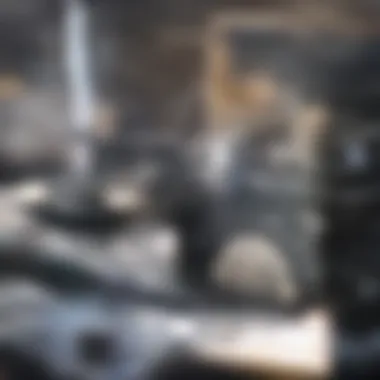
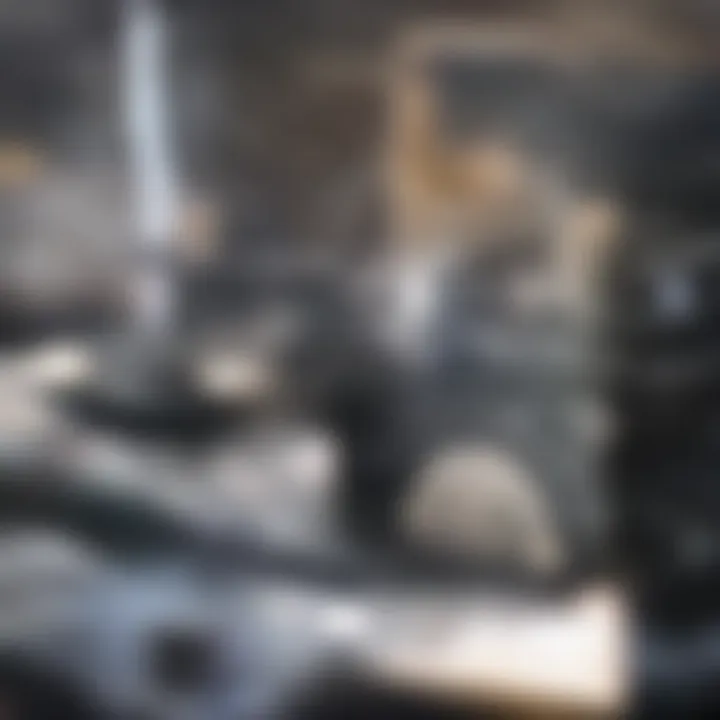
Polycrystalline Cells
Polycrystalline cells, in contrast, are made from multiple silicon crystals melted together. They are distinguished by a bluer hue and a less uniform appearance. Polycrystalline cells are generally less expensive to produce and thus are more affordable for consumers. They usually have a slightly lower efficiency than monocrystalline cells, with rates reaching around 15-20%.
Advantages:
- Lower manufacturing costs
- Better performance in high temperatures
Disadvantages:
- Relatively lower efficiency
- Requires more space to produce the same power output as monocrystalline cells
Thin-Film Cells
Thin-film cells are composed of layers of semiconductor material that are just a few micrometers thick. Their lightweight and flexible nature allows for various applications, including integration into buildings. One significant feature of thin-film technology is its ability to maintain functionality under low-light conditions. However, they generally have the lowest efficiency rates among photovoltaic types, ranging from 10-12%.
Advantages:
- Flexible and lightweight
- Lower cost of materials
Disadvantages:
- Lower overall efficiency
- Shorter lifespan compared to crystalline options
Understanding the different types of photovoltaic cells helps consumers and investors make informed decisions about solar energy systems.
A thorough knowledge of these technologies not only contributes to more efficient solar energy production but also aligns practical needs with environmental goals. Engaging with photovoltaic systems can lead to reduced energy costs and a salient contribution to a more sustainable future.
Solar Thermal Energy Systems
Solar thermal energy systems play an integral role in harnessing solar power. These systems utilize sunlight to produce thermal energy, which can be used for heating or generating electricity. This segment emphasizes several specific elements that characterize solar thermal technology, its benefits, and the essential considerations surrounding its use.
Overview of Solar Thermal Technology
Solar thermal technologies capture and convert sunlight into heat. This differs from photovoltaic systems that convert solar energy directly into electricity. The primary principle involves collecting sunlight via solar collectors, which transfers heat to a fluid, typically water or a heat-transfer fluid.
Common applications of solar thermal energy range from residential water heating to large-scale solar power plants. These systems can be broadly classified into three main types:
- Low-Temperature: Used mainly for swimming pool heating and other applications requiring modest temperature increases.
- Medium-Temperature: Employed in commercial water heating and industrial applications.
- High-Temperature: Necessary for concentrated solar power applications, which use mirrors or lenses to focus sunlight onto a small area to produce steam that drives turbines.
By capturing thermal energy, these systems provide a viable alternative to fossil fuels, directly addressing energy demand while contributing to a cleaner environment.
Key Components of Solar Thermal Systems
Several critical components are essential for the effective operation of solar thermal energy systems:
- Solar Collectors: They capture sunlight and convert it into heat. Common types include flat-plate collectors and evacuated tube collectors, each with different efficiencies and applications.
- Storage Tanks: These store the heated liquid, ensuring availability even when sunlight is not directly available.
- Pumps and Valves: They circulate the heat-transfer fluid through the system, facilitating efficient energy transfer.
- Heat Exchangers: These systems transfer thermal energy from the solar-heated fluid to the water or air intended for heating.
- Control Systems: Automated systems optimize the performance by adjusting the operation of pumps and collectors based on temperature conditions.
Understanding these components helps appreciate how solar thermal systems effectively gather and retain energy. It’s important to realize that solar thermal technology can complement other renewable energy technologies, enhancing overall energy efficiency and sustainability for both residential and commercial applications.
Solar thermal energy systems represent a strategic choice for sustainable energy production, providing substantial potential for reducing reliance on conventional energy sources.
Combination of Technologies
The combination of technologies in solar energy production plays a crucial role in enhancing efficiency and diversifying the applications of solar resources. This section explores the integration of different solar technologies, particularly photovoltaic and thermal systems. The key benefit of these hybrid systems lies in their capability to capture and utilize solar energy in multiple forms, thereby maximizing energy output and improving overall system performance. The combination of different technologies also aids in improving energy reliability, providing solutions for energy intermittency, and expanding the potential uses of solar energy.
Hybrid Systems
Hybrid systems incorporate multiple technologies to optimize the generation and use of solar energy. A notable type of hybrid system is the Photovoltaic-Thermal (PV/T) system. PV/T systems merge photovoltaic and thermal energy systems into one. This unique combination enables these systems to produce both electricity and heat from the same solar panel, making them particularly efficient.
Photovoltaic-Thermal (PV/T) Systems
Photovoltaic-Thermal systems represent a significant advancement in harnessing solar energy. These systems utilize a dual for a single installation, producing electrical energy while simultaneously collecting thermal energy through heat transfer fluids that circulate behind the photovoltaic cells. This unique feature of generating both types of energy enhances their appeal.
The primary advantage of PV/T systems is their improved efficiency. By harnessing both electricity and heat, they can achieve a much higher energy yield compared to traditional systems that focus on one method only. Furthermore, they can be particularly beneficial for applications where both electricity and heating are required, such as in residential heating systems or industrial processes. However, they also present challenges. The complexity of design and installation can lead to higher upfront costs and potential maintenance concerns.
Applications of Combined Technologies
The practicality of combined technologies can be observed in various applications.
- Residential Heating and Cooling: PV/T systems are used in homes to provide both electricity and heating for water or space, reducing reliance on other energy sources.
- Industrial Applications: Industries benefit from hybrid systems by using solar energy for both power and heat, thus lowering operational costs.
- Agricultural Uses: In agriculture, such systems can help in maintaining optimal temperature and humidity levels, while still providing energy for pumps and lights.
- Remote Areas: Hybrid systems can be pivotal in remote locations where access to the grid is limited, providing a self-sustaining energy solution.
By merging technologies, the potential for solar energy applications expands exponentially. Overall, combining different solar energy systems creates a multifaceted approach to energy production, showcasing the remarkable versatility of solar energy as a key player in the future of sustainable energy solutions.
Technological Advances in Solar Energy
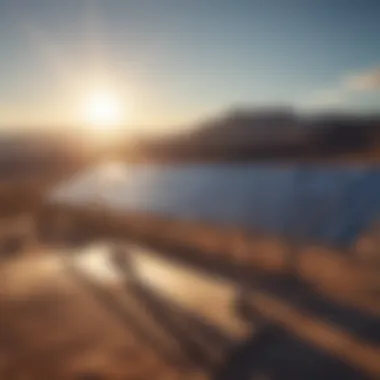
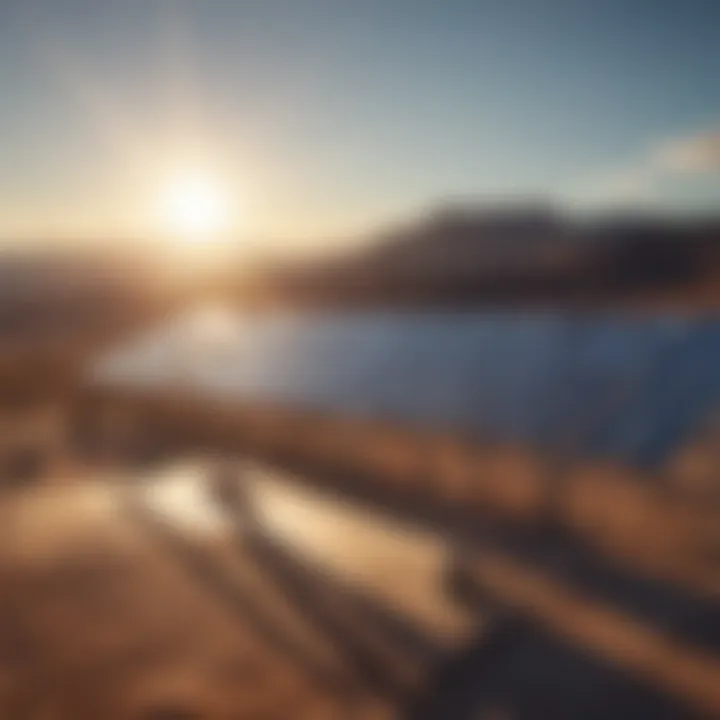
Technological advances in solar energy are crucial for the future of energy production. Innovations in this field enhance the efficiency of energy conversion and broaden the applications of solar technologies. As the demand for renewable energy sources grows, the integration of advanced technologies becomes more imperative. The continual development in solar energy helps in lowering costs and improving performance, thus making solar power a more viable option for a wider audience.
Recent Innovations
Recent innovations in solar energy technologies have revolutionized energy production. Some key developments include:
- Perovskite Solar Cells: These cells promise higher conversion efficiencies at lower production costs compared to traditional silicon cells.
- Bifacial Solar Panels: Bifacial panels capture sunlight from both sides, which can increase overall energy output.
- Flexible Solar Panels: These are lightweight and can be incorporated into various surfaces, including buildings and vehicles, making solar energy more accessible.
- Concentrated Solar Power (CSP): This technology uses mirrors or lenses to concentrate sunlight onto a small area, generating heat used to produce electricity.
These innovations are designed not only to improve efficiency but also to expand the usability of solar energy across different sectors.
Impact on Efficiency
The efficiency of solar energy systems is essential for maximizing output and minimizing costs. Technological advancements have significantly contributed to this efficiency in various ways:
- Enhanced Materials: The introduction of high-efficiency materials results in better light absorption and conversion rates. This means that more sunlight can be turned into usable energy, reducing the amount of solar panels needed.
- Improved Manufacturing Techniques: Advances in manufacturing techniques lead to fewer defects in solar panels, thereby enhancing reliability and lifespan.
- Smart Technology Integration: The use of smart technologies, including sensors and advanced monitoring systems, enables better management of energy production and consumption. This optimization allows users to harness solar energy more effectively.
Economic Context of Solar Energy
Understanding the economic context of solar energy is crucial for several reasons. As the world shifts towards renewable energy, evaluating the financial aspects surrounding solar energy production becomes more pertinent. This section will delve into the cost structures associated with solar energy and examine the impact of government incentives and subsidies.
Cost of Solar Energy Production
The cost of solar energy production has traditionally been seen as a barrier to widespread adoption. However, this perception is changing. In recent years, the average cost of solar photovoltaic systems has dropped significantly. Factors influencing these costs include:
- Technology advancements: As solar technology advances, efficiency increases, and production scales offer savings.
- Installation costs: Local labor rates and regulatory requirements can affect installation fees, but we are seeing lower rates in many regions due to increased competition.
- Material costs: The raw materials used for solar panels, such as silicon, have seen fluctuations in price due to market demands and supply chain dynamics.
As installation prices decline, the levelized cost of energy (LCOE) is becoming more competitive with fossil fuels. In some places, solar energy is now one of the cheapest forms of electricity available. This trend contributes to the growing viability of solar energy in both residential and commercial applications.
Government Incentives and Subsidies
Governments worldwide recognize the potential of solar energy to reduce carbon emissions and promote energy independence. Thus, they have introduced various incentives and subsidies to encourage both individuals and businesses to invest in solar energy. Some key components include:
- Tax credits: Many countries offer tax credits for solar panel installation, which can significantly lower the initial investment.
- Grants and rebates: Financial assistance programs can help offset installation costs, especially for those who may otherwise find the expense prohibitive.
- Feed-in tariffs: These programs guarantee that solar energy producers receive a fixed payment for the energy they generate, providing a consistent revenue stream.
According to research, government incentives can effectively boost solar adoption rates by providing the necessary financial support to overcome initial cost hurdles.
In summary, the economic context of solar energy is marked by decreasing production costs and robust governmental support. These factors make solar energy a realistic and accessible option for a wide range of consumers, contributing to the global transition towards sustainable energy solutions.
Environmental Implications
The environmental implications of solar energy production are critical to understanding its role in the contemporary energy landscape. As the world grapples with the consequences of fossil fuel dependency, solar energy emerges as a viable alternative. This section will explore two key aspects: the carbon footprint of solar energy systems and the lifecycle assessment of solar panels, illustrating their significance in promoting sustainable energy solutions.
Carbon Footprint
Solar energy is often praised for its ability to reduce greenhouse gas emissions. Unlike fossil fuels, which release high levels of carbon dioxide during combustion, solar panels generate electricity without a direct carbon output. However, it is essential to consider the entire lifecycle of solar installations. The carbon footprint encompasses the emissions attributed to the production, transportation, installation, and eventual disposal of solar panels.
While the operational phase of solar panels is carbon-free, the manufacturing process does contribute to emissions, particularly due to the extraction and processing of raw materials like silicon. Estimates suggest that the production of solar panels releases between 20-40 grams of CO2 per kilowatt-hour produced. Despite this, the overall emissions over the lifespan of a solar panel typically amount to a fraction of what traditional coal or natural gas plants would generate.
"Transitioning to solar energy is a crucial step in reducing global greenhouse gas emissions."
Therefore, using solar energy not only curtails carbon outputs during operation but also promises longer-term benefits, as the emissions incurred during manufacturing are offset by decades of clean electricity generation.
Lifecycle Assessment of Solar Panels
A lifecycle assessment (LCA) of solar panels is indispensable in grasping their environmental impact. This method evaluates the total ecological footprint from raw material extraction to the end-of-life disposal of solar panels. The assessment often considers several phases:
- Manufacturing - This phase involves raw material sourcing, energy usage in production processes, and waste generation.
- Transportation - The logistics of moving solar panels from manufacturers to installation sites can also contribute to emissions.
- Installation - The process of setting up solar arrays has its own environmental implications, which vary based on site location and methods used.
- Operation - Solar panels produce energy over a typical lifespan of 25-30 years, with minimal environmental impact during this period.
- End-of-Life Management - As solar panels reach the end of their lifecycle, recycling and disposal practices are critical factors affecting sustainability.
The conclusion drawn from LCAs indicates that solar energy systems, if managed properly, can achieve a net positive impact. Recyclability of solar materials, including metals and silicon, ensures that the environmental cost does not solely burden future generations. The sector is moving toward more efficient recycling methods, further diminishing the ecological footprint of solar energy systems.
By understanding these environmental implications, it becomes clear that solar energy is not merely a replacement for fossil fuels. It represents a shift towards a cleaner, more sustainable future. Each stage in the lifecycle of solar energy products is an opportunity for improvement as technology advances and sustainable practices become more commonplace.
Global Adoption of Solar Energy
Global adoption of solar energy is critical in understanding the advancements in energy production and consumption. As concerns about climate change and environmental sustainability grow, the push for renewable energy sources like solar has intensified. Countries worldwide are increasingly recognizing the need to transition from fossil fuels to cleaner energy alternatives. Solar energy presents a viable solution due to its abundance and decreasing costs, making it more accessible for diverse applications.
Leading Countries in Solar Energy Production
Several countries have emerged as leaders in solar energy production. Their initiatives provide valuable insights into successful practices in solar technology integration and policy-making:
- China: China dominates global solar energy production, both in terms of photovoltaic (PV) manufacturing and deployment. The government's commitment to renewable energy has fostered extensive investment in solar technologies. China also has ambitious goals for solar capacity, aiming for substantial increases in the coming years.
- United States: The U.S. has seen significant growth in solar installations, particularly in states like California, Texas, and Arizona. Policies at both federal and state levels, coupled with technological advancements and decreased costs, have facilitated this development.
- Germany: Known for its early adoption of solar technology, Germany has a robust feed-in tariff system that incentivizes residential solar panel installation. The country aims to transition completely to renewable energy sources by 2050, enhancing its commitment to sustainability.
- Japan: Following the Fukushima disaster, Japan increased investments in solar energy. The country has implemented various programs to encourage solar deployment in both residential and commercial sectors.
- India: India has set an ambitious target of 100 GW of solar energy capacity by 2022. The government's support through policy initiatives and incentives has propelled the rapid growth of solar energy in the nation.
Trends and Future Growth
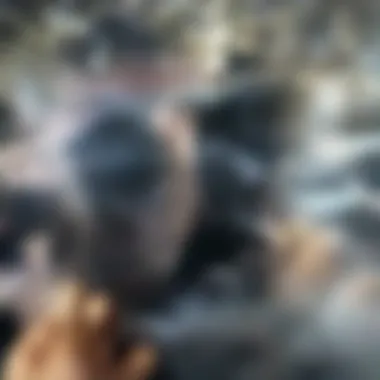
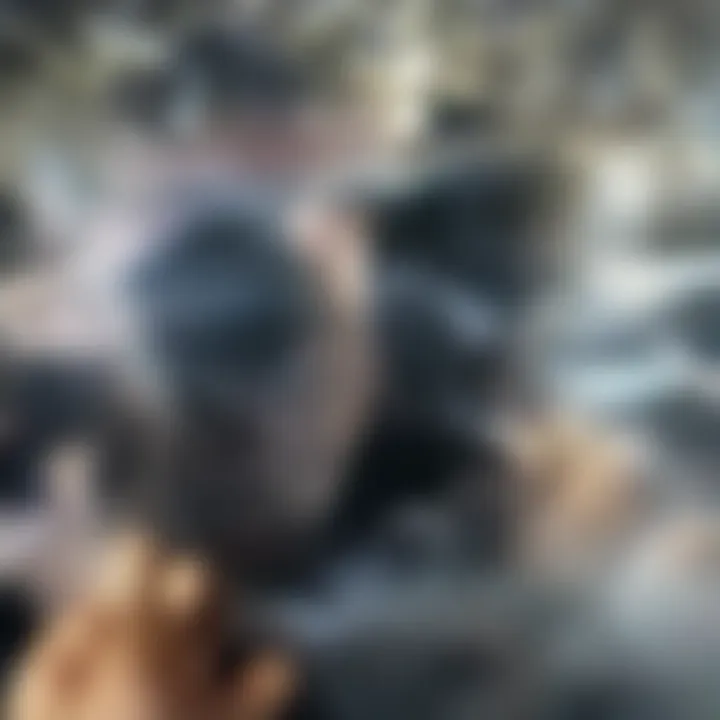
The trends and future growth in solar energy adoption are influenced by several factors. First, advancements in technology continue to increase the efficiency of solar panels, allowing for more energy output from smaller spaces. This has opened opportunities in urban environments where land may be limited.
Second, the decreasing costs of solar technology enable a wider demographic to access solar energy solutions. For instance, the price of solar panels has declined by more than 80% over the past decade. This trend helps reduce the upfront costs associated with solar installations, making it more appealing.
Additionally, countries are focusing on integrating solar energy with smart grid technologies. Smart grids help to manage energy distribution more efficiently, allowing solar energy to be utilized effectively during peak demand.
Moreover, international collaborations and investments in solar research are pivotal. These partnerships aim to improve solar technologies and share best practices, fostering global growth in solar energy adoption.
"The transition to solar energy is not just an environmental necessity but a technological and economic opportunity for nations worldwide."
The future of solar energy looks promising. Countries will continue to invest in infrastructure, policy frameworks, and technology to facilitate widespread adoption. As barriers to entry diminish, solar energy's role as a cornerstone of sustainable development becomes increasingly apparent.
Challenges in Solar Energy Production
Solar energy production represents a crucial avenue in the transition towards sustainable energy solutions. However, it is not without its challenges. Understanding these difficulties is essential for students, researchers, educators, and professionals alike. This section will delve into two primary challenges: intermittency issues and storage solutions.
Intermittency Issues
Intermittency refers to the variability inherent in solar energy generation. Solar power is reliant on sunlight, which can fluctuate due to weather conditions, time of day, and seasons. This unpredictability creates a significant challenge in solar energy production. For example, energy generation during cloudy days or at night diminishes, making it less reliable compared to fossil fuels.
The impact of intermittency affects the overall energy grid. As more solar energy is integrated, there may be times when energy production does not meet demand. This scenario necessitates effective management strategies. Utilities are tasked with balancing generation and consumption, which can lead to reliance on backup power sources, often fossil fuels.
"Solar energy's production must be matched with demand to ensure energy stability. Without effective solutions, intermittency can hamper solar adoption."
Several solutions exist to mitigate intermittency. These include:
- Forecasting Technology: Predicting solar energy generation can help utilities prepare for fluctuations.
- Diversification of Energy Sources: Incorporating a mix of energies, including wind and hydro, can stabilize supply.
- Demand Response Programs: Encouraging users to shift energy usage during peak production times can balance energy demand.
Storage Solutions
Due to the variability of solar energy, storage solutions have become a focus area for research and development. Effective storage enables excess energy generated during peak hours to be used when solar production is low. This capability is critical for a reliable solar supply.
Currently, battery storage solutions, such as lithium-ion batteries, are widely used, but they come with their own set of challenges, such as high costs and environmental concerns related to their production and disposal. There is also ongoing research to develop new technologies that can enhance energy storage efficiency and capacity. Some notable innovations include:
- Flow Batteries: These can store large amounts of energy and are ideal for long-duration storage.
- Compressed Air Energy Storage: This method uses excess energy to compress air in underground caverns, releasing it to generate electricity later.
- Thermal Energy Storage: This approach stores heat generated during the day to be used for energy production later.
While advancements are being made, finding sustainable storage solutions remains a critical area needing attention. Improving storage reliability can enhance solar energy's viability, enabling broader adoption and greater integration into the energy grid.
Future Prospects in Solar Energy Production
The future of solar energy holds significant promise as technological advancements, evolving policies, and market dynamics create new opportunities. Understanding these prospects is crucial because they reflect the trajectory of our energy landscape. As the world increasingly prioritizes sustainable energy solutions, solar power remains at the forefront due to its potential for scalability and integration within existing infrastructure.
Emerging Technologies
Emerging technologies in solar energy production are transforming how we harness sunlight. Innovations continue to enhance efficiency, durability, and cost-effectiveness of solar systems. For instance, advances in perovskite solar cells have shown great potential for higher efficiency rates at lower production costs compared to traditional silicon cells.
Moreover, the development of building-integrated photovoltaics (BIPV) is reshaping urban landscapes. These systems can be integrated directly into building materials, such as windows and roofs, thereby maximizing energy generation without requiring additional land. Another key area of innovation is solar tracking technology, which allows solar panels to follow the sun’s movement, increasing energy output by up to 25%.
In addition, bi-facial solar panels, which capture sunlight on both sides, are gaining attention for their higher energy yields. The integration of artificial intelligence (AI) into energy management systems also provides an intelligent approach to optimize energy use and maintenance of solar facilities. These developments highlight the need to monitor and adapt to new technologies that can further enhance the efficiency of solar energy production.
Integration with Smart Grids
Smart grids represent an evolution in the management of electrical grid systems, enabling efficient distribution of solar energy. Integrating solar energy systems with smart grid technology allows for improved energy management and user participation. Smart grids facilitate real-time communication between energy providers and consumers, which can optimize energy flows based on demand.
This integration can lead to more reliable energy sources due to better demand response capabilities. Consumers can utilize solar energy more effectively by participating in dynamic pricing models. When demand peaks, utility companies can incentivize users to adjust their energy consumption accordingly. Additionally, peak-shaving tactics can be employed, where excess solar energy generated during the day can be stored and released when needed to reduce strain on the grid at peak consumption times.
The synergy between solar production and smart grids may also support the implementation of electric vehicle charging stations, further driving sustainability. Such integration is key in realizing a robust solar energy ecosystem that maximizes output while minimizing waste. As technology continues to evolve, the nexus of solar and smart grid integration will play a pivotal role in shaping future energy efficiency and reliability in solar energy production.
Epilogue
In the context of this article, the conclusion serves as a critical wrap-up of the insights gathered throughout the discussion on solar energy production. This section serves several important purposes. First, it synthesizes the key themes presented in earlier sections, reinforcing the importance of understanding both solar photovoltaic and solar thermal systems as part of the larger energy landscape. By revisiting vital statistics and technological advances, readers can appreciate the evolution and current relevance of solar energy production.
Moreover, the conclusion highlights the broader implications of these energy systems. Solar energy stands as a significant player in addressing climate change and reducing reliance on fossil fuels. The discussion around economic factors, such as the costs associated with solar systems and the government incentives that encourage adoption, provides a holistic view of why solar energy is not just an alternative but a viable solution for many.
Another valuable aspect is its focus on future prospects. As the industry evolves, staying updated on emerging technologies and integration with smart grids is essential for both professionals and students alike. The conclusion emphasizes that understanding these future trends is crucial for anticipating changes in energy consumption patterns.
"Solar energy availability and technological advances present unique opportunities for achieving a sustainable future."
In summary, this section encapsulates the relevance of solar energy production in today’s context and encourages further exploration of the topic. By providing clear takeaways, it aims to enhance the reader's understanding and prompt them to engage with the material presented throughout the article.
Summary of Key Points
- Importance of Solar Energy: The discussion reinforced solar energy's role in sustainability and reducing carbon footprints.
- Technological Insights: A detailed overview of photovoltaic and solar thermal technologies was presented.
- Economic Considerations: An understanding of costs and incentives highlighted their significance in solar adoption.
- Future Technologies: Emerging tech and smart grid integration were discussed as key components of the future of solar energy.
Final Thoughts on Solar Energy
As we reflect on solar energy production, it becomes clear that this field is rich with opportunities and challenges. The blend of technology, policy, and economics makes solar energy both fascinating and vital. Not only does solar energy contribute to a more sustainable world, but it also opens doors for innovation.
Investors, researchers, and policymakers must continue to engage with this dynamic space. Bringing awareness to the advancements in solar technologies can inspire new ideas and solutions.
Ultimately, solar energy production is an ever-evolving field. It demands ongoing attention and adaptation to secure its role as a cornerstone of future energy solutions. Keeping abreast of these developments will equip all stakeholders to make informed decisions that benefit both society and the environment.